Shock in Pediatrics
Background
Worldwide, shock is a leading cause of morbidity and mortality in the pediatric population. Shock is defined as a state of acute energy failure due to inadequate glucose substrate delivery, oxygen delivery, or mitochondrial failure at the cellular level. The clinical state of shock is diagnosed on the basis of vital signs, physical examination, and laboratory data, although its recognition in the pediatric patient can be difficult.
Delay in recognizing and quickly treating a state of shock results in anaerobic metabolism, tissue acidosis, and a progression from a compensated reversible state to an irreversible state of cellular and organ damage. Morbidity from shock may be widespread and can include central nervous system (CNS) failure, respiratory failure (ie, from muscle fatigue or acute respiratory distress ssyndrome [ARDS]), renal failure, hepatic dysfunction, gastrointestinal ischemia, disseminated intravascular coagulation (DIC), metabolic derangements, and ultimately death. [1, 2, 3] (See Pathophysiology and Etiology.)
This article reviews the common physiologic foundations of shock that underpin all patients with this condition as well as defines the different pathophysiologic classifications of shock and their etiologies. The defining clinical findings of shock are described, and current diagnostic and therapeutic strategies are presented to help guide the most effective and appropriate treatment for resuscitating the child in shock. (See Pathophysiology, Presentation, Workup, Treatment, and Medication.)
Pathophysiology
Shock and shock-states are ultimately due to circulatory failure to deliver adequate substrate and remove toxins at the tissue and cellular levels.
In the nonstressed physiologic state, adequate oxygen and glucose are delivered intracellularly to mitochondria that generate 36 adenosine triphosphate (ATP) molecules per glucose molecule via aerobic metabolism and the Krebs cycle. In the stressed state in children, the ability to compensate via gluconeogenesis and glycogenolysis is limited due to small hepatic and skeletal muscle masses. Thus, glycolysis and secondary fat metabolism become the primary sources of energy substrate.
Cellular metabolism then becomes much less efficient as pyruvate is converted to lactate instead of acetyl-CoA. This shift in metabolic pathways generates only two ATP molecules per molecule of glucose and results in the accumulation of lactic acid. Ultimately, cell membrane ion pump dysfunction occurs, acidosis progresses, intracellular edema develops, intracellular contents leak into the extracellular spaces, and cell death ensues.
Key physiologic parameters that affect metabolic homeostasis include tissue blood flow, the balance between oxygen delivery and demand, and the oxygen content. Although the sufficiency of tissue oxygenation cannot be directly measured and varies by time and tissue-type, the relationship of oxygenation delivery and consumption is key to understanding the pathophysiology of shock. It is defined as follows:
VO2 = DO2 x O2 ER
Where VO2 represents oxygen consumption, DO2 represents total arterial flow of oxygen, and O2 ER is the oxygen extraction ratio (%).
In the normal state, oxygen demand is independent of delivery, as DO2 is greater than VO2. When demand increases, physiologic compensation occurs to match the need, such as through an increased heart rate and stroke volume. In shock, as DO2 declines, O2 ER increases to maintain adequate tissue oxygenation. At a critical point (DO2 crit), however, O2 ER can no longer compensate, and VO2 becomes dependent on DO2. Beyond this threshold, oxygen debt develops and blood lactate levels rise. Thus, further understanding the components of DO2 is also fundamental to the pathophysiology and treatment of shock.
Oxygen delivery
DO2 depends on the amount of blood pumped per minute, or cardiac output (CO), and the arterial oxygen content of that blood (CaO2). Thus, DO2 may be defined by the following equation:
DO2 = CaO2 × CO
The CaO2 depends on how much oxygen-carrying capacity is available, which is primarily a function of the hemoglobin (Hb) level and the arterial oxygen saturation (SaO2). A small, but typically insignificant, amount of oxygen is directly dissolved in the blood rather than bound to Hb. Therefore, CaO2 may be defined by the following formula:
CaO2 = (Hb × SaO2 × 1.34) + (0.003 X PaO2)
CO depends on the amount of blood pumped with each heartbeat, known as stroke volume (SV), and the heart rate (HR). SV depends on the ventricular end-diastolic filling volume (commonly referred to as ventricular preload), the state of myocardial contractility, and the afterload (systemic vascular resistance [SVR]) on the heart. Each of these variables affect CO and can be impaired in clinical shock states. Thus, the following relationships are observed [1] :
CO = HR × SV
SV ∝ preload, contractility, and afterload
See the image below for the factors that determine cardiac function and oxygen delivery to tissues.
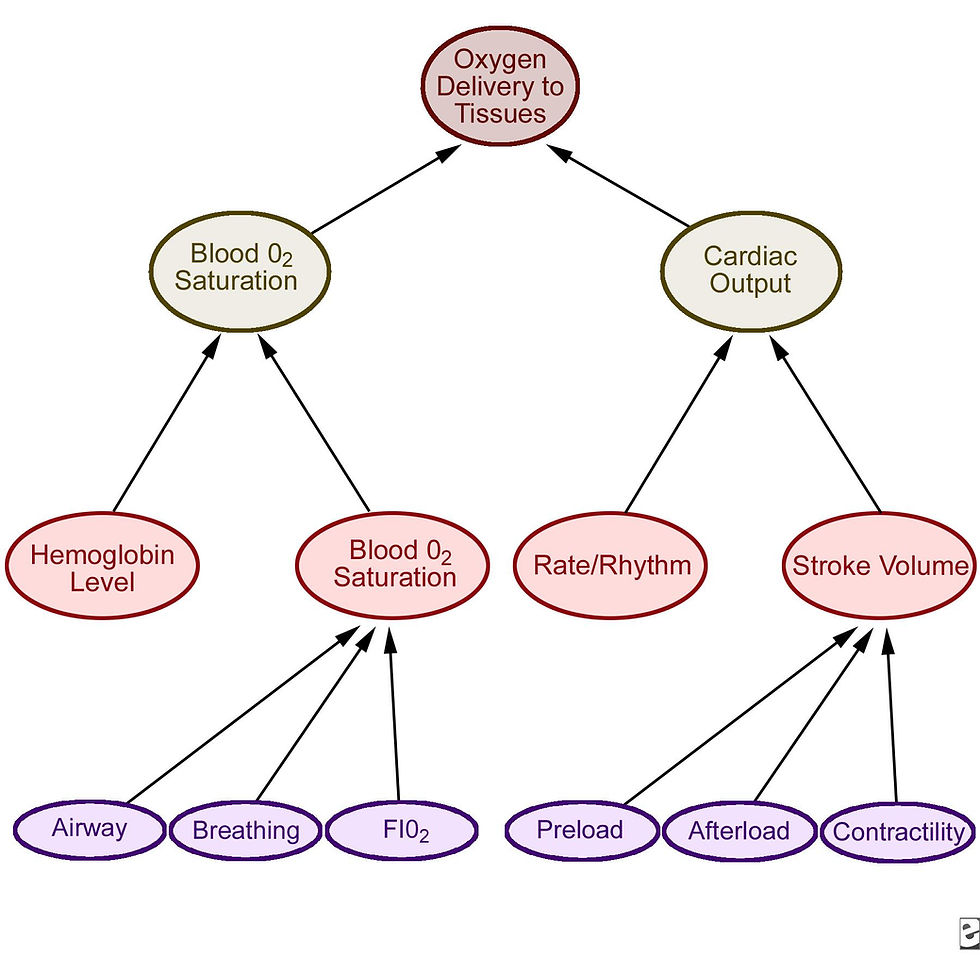
Determinants of cardiac function and oxygen delivery to tissues. FiO2 = fraction of inspired oxygen. Adapted from Strange GR. APLS: The Pediatric Emergency Medicine Course. 3rd ed. Elk Grove Village, Ill: American Academy of Pediatrics; 1998:34.
The recognition and treatment of pediatric shock is dependent on an understanding of these physiologic principles and definitions. Once understood, the different clinical presentations and causes of shock, as well as their most appropriate treatment strategies, are more easily appreciated.
Etiology
Several etiologic classifications of shock are recognized. The major categories are as follows:
Hypovolemic
Cardiogenic
Distributive
Obstructive
In each of these categories of shock, one or more of the physiologic principles that govern oxygen delivery or consumption is disturbed.
Hypovolemia leads to decreased cardiac filling, lower end-diastolic volume, and decreased stroke volume in accordance with the Frank-Starling curve and, therefore, results in decreased cardiac output. Hypovolemia due to hemorrhage additionally decreases oxygen-carrying capacity through the direct loss of available hemoglobin.
Cardiogenic shock resulting from congenital heart disease or cardiomyopathies develops from primary pump failure and inadequate cardiac output.
Distributive shock from sepsis, anaphylaxis, or high-level spinal cord injury results in peripheral vasodilation and decreased systemic vascular resistance (SVR), with venous pooling and inadequate arterial tissue perfusion to meet demand metabolic demands.
Obstructive causes of shock such as pulmonary embolism, pneumothorax, and cardiac tamponade impede either pulmonary outflow, systemic outflow, or both, thereby directly decreasing cardiac output.
Hypovolemic shock
Hypovolemic shock results from an absolute deficiency of intravascular blood volume. It is a leading cause of pediatric mortality in the United States and worldwide, although the specific causative agents may be different globally. Causes of hypovolemic shock include the following:
Intravascular volume loss (eg, from gastroenteritis, burns, diabetes insipidus, heat stroke)
Hemorrhage (eg, from trauma, surgery, gastrointestinal bleeding) (see the image below)

Hemodynamic response to shock hemorrhage model (based on normal data). Adapted from Schwaitzberg SD, Bergman KS, Harris BH. A pediatric trauma model of continuous hemorrhage. J Pediatr Surg. Jul 1988;23(7):605-9.
Interstitial loss (eg, from burns, sepsis, nephrotic syndrome, intestinal obstruction, ascites)
Gastroenteritis
Children with gastroenteritis may lose 10-20% of their circulating volume within 1-2 hours. [2] Rehydration is often impeded by concurrent vomiting, and clinical deterioration may be rapid. Common infectious causes of gastroenteritis include bacteria such as Salmonella, Shigella,Campylobacter species (spp), Escherichia coli, and Vibrio cholerae as well as viruses such as rotaviruses, adenoviruses, noroviruses, and enteroviruses. Worldwide, amebiasis and cholera are also important causes.
Trauma
In the United States, the leading cause of death in children younger than 1 year is unintentional injury. [4] A major component of traumatic death is hemorrhage. In the pediatric patient, primary sites of hemorrhage include intracranial, intrathoracic, intra-abdominal, pelvic, and external. In the pediatric patient in shock without a clear etiology and absent history, occult hemorrhage secondary to nonaccidental trauma should be considered.
Third spacing
Other causes of hypovolemia include capillary leak and tissue third spacing, which results in leakage of fluid out of the intravascular space into the interstitial tissues. Etiologies include burns, sepsis, and other systemic inflammatory diseases. Patients with such etiologies may appear edematous and overloaded with total-body fluid; however, they may be significantly intravascularly depleted, with inadequate preload, and in significant shock. Through understanding of the physiologic disturbance affecting the intravascular volume and preload, it becomes clear that such patients need additional fluid administration despite their overall edematous appearance in order to improve total arterial flow of oxygen (DO2) and to prevent or correct a state of shock.
Distributive shock
In certain clinical states such as distributive shock, normal peripheral vascular tone becomes inappropriately relaxed. Vasodilation results in increased venous capacitance, causing relative hypovolemia even if the patient has not actually had any net fluid loss. As a result, the common physiologic disturbance that affects DO2 in distributive shock is a decrease in preload caused as a result of massive vasodilation and inadequate effective intravascular volume.
Common causes of distributive shock include anaphylaxis, neurologic injury (eg, head injury, spinal shock), sepsis, and drug-related causes. [5] Causes of anaphylaxis include the following:
Medications (eg, antibiotics, vaccines, other drugs)
Blood products
Envenomation
Foods
Latex
Anaphylaxis results in mast cell degranulation with resultant histamine release and vasodilation. Neurologic injury can interrupt sympathetic input to vasomotor neurons, resulting in vasodilation. Spinal shock may result from cervical cord injuries above T1, which interrupt the sympathetic chain, allowing for unopposed parasympathetic stimulation. Such patients may present with the clinical picture of hemodynamic instability and hypotension accompanied by bradycardia, because they lose sympathetic vascular tone (resulting in vasodilation) while being unable to mount an appropriate sympathetic-mediated tachycardic response. Medications may also cause vasodilation.
Finally, sepsis causes the release of many vasoactive mediators that may produce profound vasodilation, resulting in distributive shock.
Sepsis
Sepsis may be defined as a dysregulated, systemic inflammatory state that is triggered by the presence of probable or documented infection. [6, 7]Disturbances of virtually every variable in the DO2 equation may result from the presence of infectious agents such as endotoxin or gram-positive bacterial cell wall components. Systemic molecular cascade activation leads to the release of inflammatory mediators and cytokines (eg, tumor necrosis factor–alpha [TNF-alpha]), interleukins (such as IL-1, IL-2, IL-6), products of the coagulation cascade, bradykinins, and complement activation.
Nitric oxide synthase induction results in production of the potent direct vasodilator nitric oxide, leading to inappropriate and often massive regional and systemic vasodilation. This distributive effect reduces effective preload and impairs cardiac output (CO) and DO2. Circulating toxins and inflammatory mediators can also directly depress myocardial function and reduce cardiac contractility, adding a cardiogenic component to impaired CO. Sepsis may also disrupt capillary integrity, resulting in intravascular fluid leak into tissue third spaces, causing hypovolemia. Overactivation of the clotting cascade can result in disseminated intravascular coagulation (DIC)—DIC can directly obstruct critical tissue capillary beds, resulting in microvascular obstructive shock as well as hemorrhage.
Cardiogenic shock
Impairment of cardiac contractility defines cardiogenic shock. A decreased contractile state results in decreased stroke volume (SV) and CO and, therefore, in decreased DO2. Causes of cardiogenic shock include the following:
Arrrhythmias
Cardiomyopathies/carditis: Hypoxic/ischemic, infectious, metabolic, connective tissue diseases, neuromuscular disease, toxic reaction, idiopathic
Congenital heart disease
Trauma
Iatrogenic (ie, postoperative low cardiac output syndrome)
Obstructive shock
Obstructive shock occurs when either pulmonary or systemic blood flow is impaired as a result of either congenital or acquired obstruction, leading to CO impairment and shock. Causes include acute cardiac tamponade, tension pneumothorax, massive pulmonary embolism, and other forms of pulmonary or systemic circulation obstruction such as acute or acquired pulmonary hypertension or hypertrophic cardiomyopathy. Additional causes in the neonatal period include coarctation of the aorta, interrupted aortic arch, and severe aortic valvular stenosis.
In addition to medical management for obstructive shock, treatment often depends on prompt recognition and relief of the physical obstruction, such as through pericardiocentesis for tamponade or tube thoracostomy for pneumothorax. Neonates may require maintenance of the patency of the ductus arteriosus in order to bypass the obstruction until more definitive surgery can be performed.
Epidemiology
Pediatric practitioners treating acutely ill children, from neonates to young adults, are faced with different degrees and causes of shock on a regular basis, making shock in infants and children one of the most common and, often, life-threatening conditions encountered.
The frequencies of the different etiologies of shock vary around the world by country and age.
Worlwide in 2013, 2.6 million neonates younger than 1 month died, with the most prevalent causes being neonatal encephalopathy, neonatal sepsis, congenital anomalies, and lower respiratory infections. In the same year, among children aged 1-59 months, 3.7 million children died, with the leading three causes being lower respiratory infection, malaria, and diarrheal disease. An additional 321,000 children aged 1-59 months died as a result of injury. Although these etiologies may result in death via multiple mechanisms, they suggest that sepsis from communicable diseases and hypovolemia due to infectious gastroenteritis remain major causes of shock in developing countries. [8]
In developed countries such as the United States, an estimated 37% of children presenting to pediatric emergency departments are in shock. [9] These children have a higher mortality rate compared with patients not in shock (11.4% vs 2.6%, respectively) regardless of their trauma status. Additionally, early use of the recommended Pediatric Advanced Life Support (PALS) guidelines is associated with decreased mortality (8.69% vs 15.01%, respectively) and decreased functional morbidity (1.24% vs 4.23%, respectively). [9]
Of pediatric patients who present to the emergency department in shock, sepsis is the leading cause (57%), followed by hypovolemic shock (24%), distributive shock (14%), and cardiogenic shock (5%). [10] Between 2004 and 2012, the overall incidence of sepsis/septic shock appears to have increased from 3.7% to 4.4%, although mortality has declined 10.9% over the same period. [11]
History
The clinical history of patients who present in shock is important, particularly when the etiology is not frankly evident. The etiology of shock may depend on the age of the child and the presence of any comorbid conditions.
A child with vomiting, profuse diarrhea, or both, is at risk for hypovolemic shock. A child who has experienced blunt or penetrating trauma is at risk for bleeding that may result in hemorrhagic shock. Fever may herald an infection that could result in septic shock.
All infants younger than 3 months who present in shock should be considered to be septic until proven otherwise. This concern is heightened in an immunocompromised patient who presents with fever, such as a child receiving chemotherapy or a neonate. A neonate who presents within the first weeks of life with hepatomegaly or a cardiac murmur may have a congenital obstructive ductal-dependent heart lesion that manifests as shock as the ductus arteriosus closes.
Other general, nonspecific symptoms may manifest in the child in shock. Lethargy, weakness, a sense of malaise, decreased urine output, fussiness, and poor feeding are all nonspecific symptoms that may accompany shock.
Physical Examination
The diagnosis of shock involves the clinical recognition that the body's tissues and cells are not receiving an adequate delivery of oxygen and metabolic substrates. Clinically, this relies on the detection of surrogate signs and measures and is not dictated by blood pressure.
Infants and children have a remarkable ability to preserve their central blood pressure in an attempt to protect their heart and brain in many forms of shock while critically reducing perfusion to the extremities, gastrointestinal tract, kidneys, and other end organs. As a result, shock is characterized in these patients by the following:
Tachycardia (may be absent in the hypothermic patient)
Signs of impaired organ perfusion (eg, decreased urine output, altered mental status) or delayed peripheral perfusion (eg, weak peripheral pulses, delayed capillary refill >2 sec, cool extremities)
Temperature instability (hyperthermia, hypothermia)
Tachypnea
Blood pressure: compensated versus decompensated shock
Shock can be further described by three categories: compensated, decompensated, and irreversible. To differentiate between compensated and decompensated shock, the patient’s blood pressure is compared to the American Heart Association (AHA)'s fifth-percentile systolic blood pressures for age, which are as follows [12] :
Newborn: 60 mmHg
Infant (1 mo to 1 y): 70 mmHg
Child (1 to 10 y): 70 + (2 × age [in years]) mmHg
Child older than 10 years: 90 mmHg
Children with an adequate systolic blood pressure above the normative values for age may still be in compensated shock. Through compensatory tachycardia and increase systemic vascular resistance (SVR), central perfusion to the brain and heart are still considered adequate, although other vital organ systems may be hypoperfused. If not identified and reversed, compensated shock will lead to decompensated shock.
Decompensated shock occurs when the body’s compensatory responses to shock are overwhelmed and hypotension develops. Hypotension in the pediatric shock patient is a late and ominous clinical finding. Such children, if not quickly and aggressively resuscitated, experience additional organ damage, and their condition may progress to irreversible shock, cardiovascular collapse, and cardiac arrest.
Heart rate
Because cardiac output (CO) depends on stroke volume (SV) and heart rate (HR), the body typically tries to maintain CO when SV decreases by increasing the HR. This sign is certainly not very specific in children, because children may be tachycardic from a wide variety of stimuli, including fever, pain, and agitation. Nevertheless, with the exceptions mentioned above, tachycardia is generally a fairly early and sensitive finding in compensated and decompensated shock.
Skin perfusion
In the shock state, the body also attempts to compensate by increasing SVR and shunting blood from the skin to more vital organs, such as the heart and brain. This is manifested as decreased skin perfusion, characterized by diminished distal pulses, cool skin, increased skin temperature gradient, and prolonged capillary refill. Capillary refill is best determined by pressing on a distal extremity, preferably a finger or toe, for 5 seconds and then releasing the pressure. Note the time taken to refill. At normal room temperature, the distal capillary bed normally refills within 2 seconds or less. [13]
However, patients with inappropriate vasodilation from a distributive mechanism of shock may be unable to vasoconstrict their end-organ and skin microvasculature. Therefore, in the early phases of distributive shock, such as in anaphylaxis or certain forms of sepsis, the skin may appear very briskly perfused with warm extremities, bounding pulses, and brisk capillary refill (< 1-2 sec). Thus, when distributive mechanisms of shock are present, normal skin perfusion may not be reliably reassuring. Hypotension, tachycardia, or other evidence of metabolic disturbances, such as the presence of a persistent lactic acidosis, may reinforce the recognition that tissue DO2 (total arterial flow of oxygen) is impaired.
Cold and warm shock
"Cold shock" is occasionally used to describe shock in which there are cool extremities, diminished pulses, increased skin temperature gradient, and delayed capillary refill time. Alternatively, "warm shock" is sometimes used to describe shock with warm extremities, bounding pulses, and flash capillary refill time. Although the terms are not helpful for diagnosis, they can help to guide therapeutic strategies.
Other organ system function
Mental status may reflect central perfusion to the brain. Altered mental status may coincide with profound central shock. Normal mental status may be preserved in a patient in shock if central blood pressure is adequate despite peripheral organ compromise (ie, compensated shock).
Renal perfusion may be reflected by absolute urine output. Typically, in the absence of renal damage, a well-perfused kidney can produce 1-2 mL/kg/h or more of urine. However, renal damage may result from early hypoxic-ischemic injury, causing renal tubular damage due to acute tubular necrosis (ATN) that renders urine output unreliable as an indicator of adequate intravascular volume and perfusion.
Physical findings in cardiogenic shock
Early identification of cardiogenic shock is clinically important, as treatment strategies differ from other causes of shock with great attention given to intravascular volume status and the early initiation of inotropic pharmacologic support. Physical examination findings in cardiogenic shock include the following:
Tachycardia
Hepatomegaly
Cardiac gallop
Cardiac murmurs
Precordial heave
Jugular venous distention
As a result of anatomic differences between pediatric and adult patients, evaluating infants and children for hepatomegaly is more reliable than measuring jugular venous distention, which is often difficult to appreciate in young patients.
Approach Considerations
Stabilization of the airway and breathing as well as aggressive intervention to improve the circulatory function of any patient in clinical shock always takes precedence over any further diagnostic evaluation.
After the initial stabilization, certain objective measures may help to solidify or better define the diagnosis. These measures initially may include obtaining the following laboratory studies:
Point-of-care glucose levels
Comprehensive metabolic panel (CMP)
Arterial (ABG) or venous blood gas (VBG) measurements
Serum lactate levels
Complete blood count (CBC) with differential
Prothrombin (PT) and partial thromboplastin (PTT) times
Fibrinogen and D-dimer levels
Fluid culture(s) (eg, blood, urine, cerebrospinal fluid [CSF])
Additional studies may help to identify an etiology and ultimately guide therapy of the patient in shock. Subsequent ancillary evaluation may also include the following:
Chest radiography
Mixed venous oxygen saturation
Central venous pressure measurement
Cardiac output (CO) monitoring
Near-infrared spectroscopy
B-type natriuretic peptide (BNP) levels
Serum biomarker profile
Comprehensive Metabolic Panel
A CMP may provide a wealth of information about the patient in shock. A decreased serum carbon dioxide level suggests a metabolic acidosis that may reflect a significant lactic acidosis from anaerobic metabolism associated with shock. Diarrhea also leads to direct bicarbonate loss, which may exacerbate metabolic acidosis in a patient with shock due to dehydration from diarrhea. Measurement of serum lactate levels may help to distinguish bicarbonate loss from lactic acidosis due to shock.
Hypernatremia suggests intravascular volume contraction that is consistent with hypovolemic shock. Hypoglycemia is common in shock and can rapidly be identified and treated with point-of-care testing. Elevated blood urea nitrogen (BUN) and creatinine levels and/or elevated aspartate transaminase (AST) and alanine transaminase (ALT) levels suggest secondary hypoxic-ischemic end-organ injury. Disturbed calcium homeostasis may result in low serum ionized calcium levels that can further depress myocardial function.
Blood Gas Analysis
An ABG study helps to determine the arterial oxygen tension (PaO2) of the blood, assisting in titration of supplemental oxygen delivery to the patient in shock. In addition, ABG findings help to determine the patient's acid-base status, which reflects the degree of systemic shock and the patient's response to therapy.
VBGs may also be used to determine mixed venous oxygenation saturation (discussed below).
Complete Blood Count and Coagulation Studies
In assessing the CBC, the hemoglobin (Hb) concentration is particularly important, because it determines the blood's oxygen-carrying capacity. In patients with anemia who present in severe shock, early transfusion should be considered as soon as possible.
A significantly elevated or depressed white blood cell (WBC) count, along with a WBC differential that is suggestive of infection, could support the diagnosis of septic shock. Similarly, thrombocytopenia may herald a bleeding disorder that could result in internal hemorrhage or disseminated intravascular coagulation (DIC), which may accompany septic shock. Disordered coagulation cascade activation may be further evidenced by prolonged PT and partial PTT, low fibrinogen levels, and an elevated D-dimer level.
Fluid Culture
Sepsis must be ruled out as the cause of shock, especially in children younger than 3 months, those who are immunocompromised, and those who are unvaccinated.
To exclude a serious bacterial infection, blood cultures should be obtained at presentation for all febrile children younger than 3 months. In children older than 3 months and immunocompromised patients with fever, hypothermia, leukocytosis, or other concerns for bacterial infection, blood cultures should also be obtained and sent for analysis.
CSF and urine cultures should also be considered as dictated by the child's clinical presentation and host risk factors.
Chest Radiography
Evaluation of the cardiac silhouette on a chest radiograph may help to delineate cardiogenic shock, which may feature cardiomegaly (see the image below), from hypovolemic shock, in which the heart size appears small.

Chest radiograph in a patient with cardiomegaly, which may accompany cardiogenic shock
The chest radiograph may also reveal signs of pneumonia or other pulmonary disorders. Respiratory distress in a patient in shock may result from acute respiratory distress syndrome (ARDS), which may develop in any patient in shock or result from pneumonia and sepsis.
Mixed Venous Oxygen Saturation
Mixed VBG analysis can be utilized to determine the venous Hb oxygen saturation, or it can be directly measured by co-oximetry. A true mixed venous sample (SvO2) from the pulmonary artery may be obtained from the distal port of a Swan-Ganz catheter—however, these catheters are infrequently utilized in pediatric patients. More often, the oxygen saturation of a sample of mixed central venous blood (ScvO2) returning to the heart that is taken from a central venous catheter can be used as an SvO2 surrogate.
By comparing the mixed venous oxygen saturation (ie, SvO2) with the arterial oxygen saturation (SaO2), the arteriovenous oxygen saturation difference and oxygen extraction ratio (O2 ER) can be determined. In a patient with a relatively normal SaO2 (93-100%), the normal SvO2 is 65-77%, as the tissues typically extract 23-35% of oxygen delivered to them. If the oxygen extraction difference is greater than 35%, perfusion to the tissue capillary beds may be inadequate, reflecting a state of shock. Alternatively, if the oxygen extraction difference is less than 23%, oxygenated blood may be shunting past tissue capillary beds as a result of inappropriate distribution of blood flow (ie, distributive shock, with arteriovenous shunts resulting from vasodilation). Sepsis can also inhibit the cellular metabolic machinery, decreasing oxygen extraction and leading to an increase in venous saturation.
Central Venous Pressure
A central venous catheter in the superior vena cava may transduce the pressure generated by the blood in that vessel, the central venous pressure (CVP), which represents the back-pressure into the systemic venous circulation. Care must be taken to not rely entirely on such measurements. The cardiac filling pressure measured by these catheters reflects ventricular function and compliance—not necessarily intravascular volume alone. Nevertheless, such values, taken in context with the clinical examination findings, may help to determine the patient's clinical status. A normal CVP in a normal, compliant heart is typically 8-12 cm H2 O. Higher pressures may reflect volume overload or poor right-sided heart compliance or function.
Cardiac Output Monitoring
Classically, the cardiac index (CI) is determined by pulmonary artery catheter measurement of the CO, although pulmonary artery catheterization is now infrequently done in pediatric patients. Instead, many innovative invasive or noninvasive surrogate technologies for CO determination are increasingly available and utilized in this patient population. These include Doppler echocardiography, the pulse contour cardiac output (PiCCO) catheter, and the femoral artery thermodilution catheter (FATD).
The CI is calculated by dividing the CO by the body surface area (BSA). Normal CI is 3.5-5.5 L/min/m2, and values between 3.3 and 6 L/min/m2 have been associated with optimal outcomes from pediatric septic shock. [14, 15] Monitoring changes in CI during intravascular volume or cardiotropic infusions may help to guide and optimize administration of these therapies.
Near-Infrared Spectroscopy
A relatively new technology currently used in many pediatric intensive care units (PICUs) is near-infrared spectroscopy (NIRS). [16, 17, 18] An optical probe placed over a patient's skin, such as the forehead, the flank over the kidneys, or the abdomen, sends an infrared signal through the skin and measures the pooled-tissue oxygen saturation. Because most of the blood in any given region is predominantly venous, the oxygen saturation is close to that of the tissue venous oxygen saturation in that region. This allows for a noninvasive measurement and an observation of the trend of an increased or decreased tissue oxygen saturation to be followed in critical tissue beds, such as the brain, kidneys, or mesenteric region. Such information may help to identify adequate or inadequate oxygen delivery. It is analogous to determining SvO2 and may help to guide evaluation and response to therapy.
B-Type Natriuretic Peptide
BNP is a hormone produced by ventricular myocytes that is released in response to myocardial wall stress. In adult and pediatric studies, plasma BNP levels have been shown to be elevated in sepsis and in congestive heart failure with cardiogenic shock. Elevated levels of BNP reflect myocardial stress, and improvement in cardiac function is associated with normalization of BNP levels.[19, 20]
Sepsis Biomarker Risk Model
Studies in recent years have focused on identification of serum protein biomarkers which may serve as prognostic indicators for pediatric sepsis. One such model, the PEdiatRic SEpsis biomarkEr Risk modEl (PERSEVERE), includes five proteins: C-C chemokine ligand 3 (CCL3), heat shock protein 70 kDa 1B (HSPA1B), interleukin-8 (IL-8), elastase 2 (ELA2), and lipocalin 2 (LCN2). This model estimated mortality with a sensitivity of 83% and a specificity of 75%. [21,22]
Shock in Pediatrics Treatment & Management
Approach Considerations
Early goal-directed therapy (EGDT) is targeted at maintaining and restoring an adequate airway, oxygenation, ventilation, and circulation within the first hour of shock onset. Adequate circulation is further defined by adequate perfusion, normal blood pressure for age, and normal or threshold heart rate.
As the initial principles of shock treatment are largely the same regardless of etiology, the 2007 American College of Critical Care Medicine (ACCM) clinical practice parameters for the treatment of pediatric and neonatal septic shock are appropriate to utilize for the initial treatment of pediatric shock. [14]
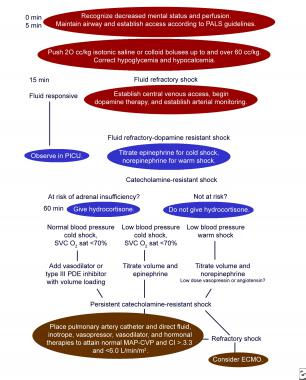
Pediatric shock management algorithm. ACTH = adrenocorticotropic hormone; CI = cardiac index; ECMO = extracorporeal membrane oxygenation; MAP-CVP = mean arterial pressure-central venous pressure; PALS = Pediatric Advanced Life Support; PDE = phosphodiesterase; PICU = pediatric intensive care unit; SVC O2 = superior vena cava oxygen saturation.
Additionally, the 2012 Surviving Sepsis Campaign update provides supplemental considerations for pediatric shock management. [15]
Appropriate therapeutic goals for the treatment of pediatric shock should, therefore, include the following:
Normal mental status
Normal blood pressure for age
Normal or threshold heart rate for age
Normal and equal central and peripheral pulses
Warm extremities with capillary refill of 2 seconds or less
Urine output greater than 1 mL/kg/h
Normal serum glucose levels
Normal serum ionized calcium levels
Decreasing serum lactate levels
As an additional therapeutic goal, the ACCM guidelines also recommend resuscitating children with septic shock to a central venous oxygen saturation (ScvO2) of more than 70%. [14] One randomized controlled trial of EGDT in septic children that compared continuous monitoring ScvO2 to unmonitored ScvO2demonstrated a significant reduction in 28-day mortality (from 39.2% to 11.8%) and fewer new organ dysfunctions. [23] A follow-up prospective cohort study also compared intermittent ScvO2 monitoring versus no monitoring in children with fluid refractory shock; it further showed a 39% decrease in mortality and improved organ dysfunction. [24] These studies support the use of ScvO2monitoring and EGDT to guide resuscitation beyond basic clinical measures, such as vital signs, central venous pressure (CVP), and urine output alone.
Initial Resuscitation
Regardless of the cause of shock, the ABCs (airway, breathing, circulation) must be immediately evaluated and stabilized without delay for further diagnostic workup or imaging studies. Place the patient on appropriate noninvasive monitors, such as a pulse oximeter and cardiorespiratory monitor.
The patient's airway must be patent, and the patient must be adequately oxygenated and ventilated. Initially, administer 100% supplemental oxygen at a high flow rate via a face mask or, if respiratory distress is present, via high flow nasal cannula or noninvasive continuous positive airway pressure (CPAP). If the patient is in respiratory failure, consider intubating and providing mechanical ventilation. If the airway can be maintained and oxygenation supported without immediate intervention, delay intubation to allow for initiation of aggressive fluid resuscitation. This is recommended because of the negative—and potentially catastrophic—effect of positive pressure ventilation on venous return and cardiac stability in the hypovolemic patient.
Once the airway has been stabilized and adequate ventilation and administration of oxygen have been ensured, immediately focus on improving circulation and systemic oxygen delivery. Circulatory improvement is achieved via volume expansion and, if necessary, pharmacologic therapy with vasopressors and cardiac inotropic agents.
Glucose and Calcium Stabilization
Children in shock, particularly when due to sepsis, are at risk for both hypoglycemia and hypocalcemia. These conditions should be rapidly identified and corrected to improve survival and minimize morbidities.
Hypoglycemia
Hypoglycemia is common as a result of inadequate glycogen stores, increased glucose consumption, and metabolic failure. Neonates and infants have limited glycogen stores, which may become rapidly depleted during shock and lead to hypoglycemia. Alternatively, high levels of endogenous and exogenous catecholamines may cause a relative insulin-resistant state that can result in serum hyperglycemia.
Because glucose is the major metabolic substrate, a rapid bedside glucose test should be performed on all patients who present in shock. If the glucose level is low, replacement therapy is provided with intravenous (IV) dextrose. The dose of dextrose is 0.5-1 g/kg. It can be given as follows:
5-10 mL/kg of D10W
2-4 mL/kg of D25W
1-2 mL/kg D50W
Following correction of hypoglycemia, if oral intake is contraindicated, a continuous dextrose infusion is recommended as part of maintenance IV fluids for pediatric patients.
Hypocalcemia
Shock may also cause alterations in available levels of serum ionized calcium, despite normal total serum calcium levels. Hypocalcemia in the shock state is due to impaired parathyroid hormone function, decreased hepatorenal vitamin D hydroxylation, and end-organ resistance. Furthermore, administered blood products (which contain citrate) may bind free available calcium, thereby additionally decreasing the available ionized calcium levels.
Calcium mediates excitation-contraction coupling in muscle cells, including cardiac muscle; low levels of ionized calcium have been shown to be associated with cardiac dysfunction and more severe organ dysfunction. [25] The availability of functioning ionized calcium also depends on the patient's serum acid-base status; an acid environment favors the dissociation of calcium from proteins, making it available as a cofactor in cell function. Calcium is also indicated for treating shock caused by arrhythmias that are precipitated by hyperkalemia, hypermagnesemia, or calcium channel blocker toxicity.
Calcium may be provided either as calcium gluconate or calcium chloride. However, calcium chloride has been shown to produce higher and more consistent levels of available calcium and, therefore, is recommended in the acute resuscitation of a child in shock. [26] The recommended calcium chloride (10%) dose is 10-20 mg/kg (0.1-0.2 mL/kg) IV, administered at an IV infusion rate that does not exceed 100 mg/min. Further therapy may be guided by repeat plasma ionized calcium measurements. Calcium should not be given empirically during active cardiopulmonary resuscitation (CPR) without clear indication because this has been associated with increased mortality. [27]
Fluid Resuscitation
The major physiologic abnormality in most forms of pediatric shock is either an absolute or a relative intravascular hypovolemia. Children with hypovolemic shock who receive appropriate aggressive fluid resuscitation within the first hour of resuscitation have the most optimal chance of survival and recovery. [28,29] In one study, a higher mortality rate was demonstrated in children who received less than 40 mL/kg of fluid resuscitation in the first hour and those whose treatment was not initiated within the first 30 minutes after the diagnosis.[30] Therefore, the therapy of choice is rapid and aggressive fluid resuscitation.
If possible, place a minimum of two large-bore, free-flowing IV catheters. If vascular access is not easily and readily achieved, then an intraosseous (IO) needle may be placed into the bone marrow for rapid fluid administration. Such an IO line can be considered as good as an IV line for the purpose of any fluid or medication administration that is necessary for the acute resuscitation of a compromised infant or child in shock. [31]
Administer 20 mL/kg of an isotonic crystalloid infusion, such as 0.9% isotonic sodium chloride or lactated Ringer solution, over 5 minutes or less. This may be most rapidly achieved by a disconnect-reconnect technique using large volume syringes. In this technique, one provider prepares syringes of normal saline or lactated Ringer solution while the other pushes the fluid filled syringe into an IV or IO catheter. [32]
As soon as the initial bolus of fluid (20 mL/kg) has been infused, reevaluate the patient. If the patient retains the clinical appearance of shock, immediately infuse another 20 mL/kg of fluid and repeat the cycle. Additional boluses are titrated to clinical improvement with improved mental status, hemodynamics, perfusion, and urinary output. Ultimately, a child with severe hypovolemia or sepsis should receive 60 mL/kg of volume in the first 15 minutes of early goal-directed therapy (EGDT).
If more than 2-3 volumes of crystalloid have been infused into a patient at risk for hemorrhage (eg, from trauma), administer blood or packed red blood cells (PRBCs). If rales or hepatomegaly develop at any point during the volume resuscitation, the resuscitation should transition from volume administration to inotropic therapy initiation.
Precautions
Cardiogenic shock
An exception to repetitive volume resuscitation in a child with shock is the child who presents with cardiogenic shock. During the initial infusion of fluid for volume expansion, the child can be evaluated for the possibility of cardiogenic shock. If the cause of shock is cardiogenic in etiology, judicious fluid boluses of 5-10 mL/kg should be utilized and balanced with the potential need for early inotropic support to prevent fluid overload.
Global management variation
Of note, a large study in African children presenting with apparent sepsis to resource-limited facilities demonstrated a worse outcome for children treated with what would be considered standard fluid resuscitation by Western practice. These results should be considered when treating children in the region of the world studied. The authors suggest that further study is warranted regarding volume expansion and fluid effects on septic children in different settings and with various etiologies. [33]
Stratification of initial mortality risk
Providing additional insight into the role of fluid status, one large study in the United States showed that when stratified for mortality risk, increased volume fluid resuscitation and positive fluid balance in patients with a low initial mortality have worse outcomes, with persistence of multisystem organ failure and death. Patients with a moderate to high initial mortality risk did not show increased mortality with aggressive fluid management. [34]
Antibiotics and Source Control
If septic shock is a concern, initial coverage with empiric antibiotics is essential to eliminating the precipitating cause of shock. The current standard of care is to initiate empiric antibiotics within the first hour of the diagnosis of severe sepsis. Delayed antimicrobial therapy, specifically greater than 3 hours after recognition of sepsis, has been associated with increased mortality and prolonged organ dysfunction. [35]
Blood cultures should be obtained before antibiotic administration if possible, or as soon as clinical stability permits. Early source control is also recommended.
Management of significant septic shock should be multidisciplinary and should involve the resources of infectious disease specialists when available. [15, 36, 37]The choice of antibiotics may vary, depending on the patient’s age, comorbidities, epidemiologic disease prevalence, and previous antibiotic exposures. Neonates are often started on a combination of ampicillin and gentamicin. Older infants and children may be covered with a third-generation cephalosporin, possibly along with expanded gram-positive organism coverage with vancomycin initially. Intravenous immunoglobulin (IVIG) has not shown any benefit in treating pediatric patients with septic shock. [38]
Due to the global burden caused by septic shock, multiple international groups have worked to produce sepsis guidelines, bundles, and checklists, including The Surviving Sepsis Campaign [15] and the World Federation of Pediatric Intensive and Critical Care Societies. [39]
Blood Therapies
During the resuscitation of the pediatric patient in shock with a central venous oxygen saturation (ScvO2) that is less than the goal of 70%, transfusion of packed red blood cells (PRBCs) to a threshold of 10 g/dL may be beneficial to increase arterial blood oxygen content (CaO2).
In the hemodynamically stable pediatric patient with resolving shock, a similar restricted transfusion threshold of 7 g/dL (as in adults) is targeted. If the cause of shock is hemorrhage from trauma, then ongoing bleeding may need to be surgically addressed.
Vasoactive Agents
Following the initial fluid resuscitation, if shock persists, then it is described as fluid-refractory shock. In this situation, the early initiation of inotropic catecholamine infusions is recommended to potentially help restore sufficient total arterial flow of oxygen (DO2) through improved perfusion and cardiac function.
Initial administration of inotropic agents via peripheral vascular access is recommended until central venous access is obtained. The specific inotropic agent used depends on the hemodynamics, cardiac output (CO), and systemic vascular resistance (SVR) of the patient in shock. For the patient in cold shock with high SVR, dopamine and epinephrine are first-line agents. For the patient with warm shock and low SVR, norepinephrine is recommended. Based on clinical measures to include the central venous oxygen saturation (ScvO2) and potentially directly or indirectly measured cardiac index (CI), vasodilators such as milrinone may also be used to treat low cardiac output states.
For the patient with persistent, catecholamine resistant shock despite appropriate volume and blood and electrolyte repletion, conduct additional evaluation to rule out alternate causes. Pericardial effusion, pneumothorax, and pulmonary embolism all should be ruled out. Any malignant arrhythmias should be converted to normal sinus rhythm as soon as possible. Consideration of a potential endocrine emergency, such as relative/absolute adrenal insufficiency or hypothyroidism is also necessary.
Supportive Therapies
Corticosteroids
The use of corticosteroids in shock, particularly septic shock, is controversial. Many large-scale, controlled trials in animals and humans have not demonstrated improved outcome with corticosteroid use. [40, 41, 42]Nevertheless, a question remains as to whether patients in severe septic shock have adequate levels of circulating glucocorticoids to support their physiology when it is severely stressed.
Adrenocortical failure or infarction, known as Waterhouse-Friderichsen syndrome, may result in cardiovascular failure and hyporesponsiveness to catecholamines. In affected patients, initiation of stress-dose hydrocortisone, in the range of 50-100 mg/m2/day IV, may be beneficial and lifesaving. A serum cortisol level may be drawn prior to initiating the first dose of corticosteroids, and if this random serum cortisol level is low, then replacement doses may be beneficial. Moreover, some data suggest a potential role for corticosteroid replacement therapy in select patients with septic shock.
Furthermore, select patients may have adrenal insufficiency, rendering them fluid refractory and catecholamine resistant during resuscitation from shock. Some practitioners evaluate a baseline serum cortisol level in children with fluid-refractory, catecholamine-resistant shock and/or perform a corticotropin stimulation test with 250 mcg of corticotropin, and then treating the patient with hydrocortisone, although the use of such serum measurements has not been shown to result in improved outcomes.
Therapy is continued for patients who prove to have an absolute baseline cortisol level of less than 20 mcg/dL and/or a depressed response to the corticotropin stimulation test (ie, a rise of < 9 mcg/dL at 30 and 60 minutes after administration of corticotropin). [43]
Bicarbonate
Sodium bicarbonate use in the treatment of shock is also controversial. During shock, acidosis develops, which impairs myocardial contractility and optimal function of catecholamines. However, treatment with bicarbonate may worsen intracellular acidosis while it corrects serum acidosis. This occurs because bicarbonate is an ion that does not readily traverse semipermeable cell membranes. Hence, bicarbonate combines with acid in serum, resulting in the production of carbon dioxide and water, as defined by the Henderson-Hasselbalch equation.
If the increased carbon dioxide is not removed via ventilation, it readily enters the cell and drives the Henderson-Hasselbalch reaction in the opposite direction, thereby increasing intracellular acidosis. Worsened myocardial intracellular acidosis may result in a decrease in myocardial contractility. [44] In addition, bicarbonate administration may result in hypernatremia and hyperosmolality, thereby decreasing the availability of ionized calcium.
Finally, laboratory and clinical data have not demonstrated that bicarbonate administration improves the ability to defibrillate, improves total arterial flow of oxygen (DO2), or improves survival rates in shock and cardiac arrest. [45, 46]Studies in patients with cardiovascular arrest have not demonstrated improved survival rates associated with the use of bicarbonate. Thus, acidosis that results from shock should ideally be corrected with increased perfusion from volume supplementation and judicious use of inotropic medications in conjunction with optimal ventilation.
Other Special Considerations
Other modalities of supportive care and multiple system organ support may be required. Lung-protective modes of ventilation should be utilized, such as allowing for permissive hypercapnia, low tidal volumes, and limiting peak plateau pressures. Nutrition should be optimized with early enteral feeds in children who will tolerate them and via IV nutrition in those who are unable to tolerate enteral feeds. Glycemic control should target glucose levels of 180 mg/dL or less. Fluid overload should be limited and reversed following the resolution of shock which may require diuretics and, potentially, renal replacement therapies such as continuous veno-venous hemofiltation (CVVH) or intermittent dialysis., For patients with refractory shock, extracorporeal membrane oxygenation (ECMO), if available, should be initiated. [47, 48, 49]
All of the therapies discussed here are aimed at restoring adequate perfusion to the tissues and organs of the body as soon as possible. Ongoing support offers the body the opportunity to repair the hypoxic and ischemic damage sustained, with the ultimate goal of functioning, intact patient survival.
Medication Summary
A variety of therapeutic treatments may be required for pediatric patients in shock. Possible therapies may include the following:
Inotropic medications
Dextrose
Electrolytes and calcium stabilization
Prostaglandin E1
Corticosteroids
Inotropic agents
Class Summary
Inotropic agents increase myocardial contractility and have variable effects on peripheral vascular resistance. First-line inotropic agents in pediatric shock include dopamine and epinephrine. On the basis of cardiac output (CO) and systemic vascular resistance (SVR), other vasoactive agents indicated in the treatment of shock may include vasoconstrictors (eg, norepinephrine, phenylephrine) or vasodilators (eg, dobutamine, milrinone). [50, 51] Which agents are indicated and which are effective in patients with any given etiology of shock depends on the end-diastolic volume and contractile state of the patient's cardiovascular system.
Dopamine
Dopamine is used for refractory hypotension following adequate volume resuscitation. It stimulates beta1- and alpha1-adrenergic and dopaminergic receptors in a dose-dependent fashion. In low doses, dopamine acts on dopaminergic receptors in renal and splanchnic vascular beds, causing vasodilatation in these beds. In midrange doses, it acts on beta-adrenergic receptors to increase heart rate and contractility, improve cardiac output, and enhance conduction (increasing sinoatrial rate) in the heart. In high doses, it acts on alpha-adrenergic receptors to increase systemic vascular resistance and raise blood pressure.
Dobutamine
Sympathomimetic agent with primarily beta1-agonist effects, increasing heart rate and blood pressure. Some weak beta2-mediated peripheral vasodilation. Little effect on alpha receptors. May precipitate ventricular dysrhythmias, although potentially less likely to do so than epinephrine.
Epinephrine (Adrenalin)
Epinephrine is used for hypotension refractory to dopamine. Its alpha-agonist effects include increased peripheral vascular resistance, reversed peripheral vasodilatation, systemic hypotension, and vascular permeability. Its beta2-agonist effects include bronchodilation, chronotropic cardiac activity, and positive inotropic effects.
Norepinephrine (Levophed)
Norepinephrine is used for protracted hypotension following adequate fluid-volume replacement. This agent stimulates beta1- and alpha-adrenergic receptors, thereby increasing cardiac muscle contractility and heart rate, as well as vasoconstriction.
Phenylephrine
Strong alpha-receptor stimulant with little beta-adrenergic activity that produces vasoconstriction of arterioles in the body, helping increase systemic vascular resistance.
Phosphodiesterase Enzyme Inhibitor
Class Summary
Milrinone is the primary phosphodiesterase (PDE) inhibitor used in pediatrics, and it works via a different mechanism than that of the catecholamines. Milrinone inhibits PDE III, producing an increase in intracellular cyclic adenosine monophosphate (cAMP), which raises intracellular calcium levels and thereby improving cardiac inotropy and peripheral vasodilation.
Milrinone may be used together with catecholamines to further increase myocardial contractility while reducing systemic vascular resistance (SVR) and afterload. It can be useful in improving perfusion in patients who remain in compensated shock with poor peripheral perfusion but a normal central blood pressure and adequate intravascular volume. Milrinone is also often a useful adjunct in patients who have low cardiac output syndrome following congenital heart corrective surgeries. [52]
Adverse effects of milrinone may include arrhythmias and thrombocytopenia. [53,54] Care must be taken when choosing to start phosphodiesterase inhibitors because of their vasodilator effects and their long half-life.
Milrinone
Milrinone is a selective PDE III inhibitor that acts as a positive inotrope and vasodilator with little chronotropic activity.
Prostaglandins, Endocrine
Class Summary
Neonates who present with shock associated with a large liver, enlarged cardiac silhouette, or heart murmur may have obstructive shock that develops because of closing of the ductus arteriosus. Prior to closure, the ductus arteriosus allows sufficient systemic blood flow to bypass the obstructive lesion. In patients in whom the ductus arteriosus has closed, initiation of prostaglandin E1 to maintain or reestablish its patency may be lifesaving. Adverse effects may include fever, apnea, or hypotension due to vasodilation. Evaluation of cardiac anatomy with echocardiography, performed by a pediatric cardiologist, should be obtained as soon as possible.
Alprostadil IV (Prostin VR)
Alprostadil is a synthetic prostaglandin E1 and induces vascular smooth muscle and ductus arteriosus vasodilation. This agent is beneficial in infants with congenital defects that restrict pulmonary or systemic blood flow and in patients who depend on a patent ductus arteriosus for adequate oxygenation and perfusion.
Corticosteroid
Class Summary
The use of corticosteroids in shock is controversial. However, it is possible that patients in severe septic shock have inadequate levels of circulating glucocorticoids and may benefit from stress dosing of steroids.
Hydrocortisone (Solu-Cortef, Cortef)
Hydrocortisone is a glucocorticoid with complex and varied metabolic effects when given systemically. It is the corticosteroid of choice in vasopressor-refractory shock because of its mineralocorticoid activity and glucocorticoid effects.
References
Epstein D, Randall CW. Cardiovascular physiology and shock. Nichols DG, ed. Critical Heart Disease in Infants and Children. 2nd Ed. Philadelphia, PA: Mosby Elsevier; 2006. 17-72.
Nadel S, Kissoon N, Ranjit S. Recognition and Initial Management of Shock. Nichols DG, ed. Roger’s Textbook of Pediatric Intensive Care. Philadelphia, PA: Lippincott, William & Wilkins; 2008. 372-383.
Smith LS, Hernan LJ. Shock states. Fuhrman BP, Zimmerman J, eds. Pediatric Critical Care. 4th Ed. Philadelphia, PA: Elsevier Saunders; 2011. 364-378.
American Academy of Pediatrics. Policy statement--child fatality review. Pediatrics. 2010 Sep. 126(3):592-6. [Medline].
[Guideline] Simons FE, Ardusso LR, Dimov V, Ebisawa M, El-Gamal YM, Lockey RF, et al. World Allergy Organization Anaphylaxis Guidelines: 2013 update of the evidence base. Int Arch Allergy Immunol. 2013. 162(3):193-204. [Medline].
Ackerman AD, Singhi S. Pediatric infectious diseases: 2009 update for the Rogers' Textbook of Pediatric Intensive Care. Pediatr Crit Care Med. 2010 Jan. 11(1):117-23. [Medline].
Goldstein B, Giroir B, Randolph A. International pediatric sepsis consensus conference: definitions for sepsis and organ dysfunction in pediatrics. Pediatr Crit Care Med. 2005 Jan. 6(1):2-8. [Medline].
Global, regional, and national age-sex specific all-cause and cause-specific mortality for 240 causes of death, 1990-2013: a systematic analysis for the Global Burden of Disease Study 2013. Lancet. Jan 2015. 385:117-71. [Medline].
Carcillo JA, Kuch BA, Han YY, Day S, Greenwald BM, McCloskey KA, et al. Mortality and functional morbidity after use of PALS/APLS by community physicians. Pediatrics. 2009 Aug. 124(2):500-8. [Medline].
Fisher JD, Nelson DG, Beyersdorf H, Satkowiak LJ. Clinical spectrum of shock in the pediatric emergency department. Pediatr Emerg Care. 2010 Sep. 26(9):622-5. [Medline].
Balamuth F, Weiss SL, Neuman MI, Scott H, Brady PW, Paul R, et al. Pediatric severe sepsis in U.S. children's hospitals. Pediatr Crit Care Med. 2014 Nov. 15(9):798-805. [Medline]. [Full Text].
American Heart Association. Part 2: Systematic approach to the seriously ill or injured child. Chameides L, Samson RA, Schexnayder SM, Hazinski MF, eds. Pediatric Advanced Life Support Provider Manual. Dallas, TX: American Heart Association; 2011.
Carcillo JA. Capillary refill time is a very useful clinical sign in early recognition and treatment of very sick children. Pediatr Crit Care Med. 2012 Mar. 13(2):210-2. [Medline].
[Guideline] Brierley J, Carcillo JA, Choong K, Cornell T, Decaen A, Deymann A, et al. Clinical practice parameters for hemodynamic support of pediatric and neonatal septic shock: 2007 update from the American College of Critical Care Medicine. Crit Care Med. 2009 Feb. 37(2):666-88. [Medline].
[Guideline] Dellinger RP, Levy MM, Rhodes A, Annane D, Gerlach H, Opal SM, et al. Surviving sepsis campaign: international guidelines for management of severe sepsis and septic shock: 2012. Crit Care Med. 2013 Feb. 41(2):580-637. [Medline].
Ferrari M, Mottola L, Quaresima V. Principles, techniques, and limitations of near infrared spectroscopy. Can J Appl Physiol. 2004 Aug. 29(4):463-87. [Medline].
Adcock LM, Wafelman LS, Hegemier S, Moise AA, Speer ME, Contant CF, et al. Neonatal intensive care applications of near-infrared spectroscopy. Clin Perinatol. 1999 Dec. 26(4):893-903, ix. [Medline].
Ghanayem NS, Wernovsky G, Hoffman GM. Near-infrared spectroscopy as a hemodynamic monitor in critical illness. Pediatr Crit Care Med. 2011 Jul. 12(4 Suppl):S27-32. [Medline].
Post F, Weilemann LS, Messow CM, Sinning C, Münzel T. B-type natriuretic peptide as a marker for sepsis-induced myocardial depression in intensive care patients. Crit Care Med. 2008 Nov. 36(11):3030-7. [Medline].
Domico M, Liao P, Anas N, Mink RB. Elevation of brain natriuretic peptide levels in children with septic shock. Pediatr Crit Care Med. 2008 Sep. 9(5):478-83. [Medline].
Wong HR, Salisbury S, Xiao Q, Cvijanovich NZ, Hall M, Allen GL, et al. The pediatric sepsis biomarker risk model. Crit Care. 2012 Oct 1. 16(5):R174. [Medline]. [Full Text].
Wong HR, Weiss SL, Giuliano JS Jr, Wainwright MS, Cvijanovich NZ, Thomas NJ, et al. Testing the prognostic accuracy of the updated pediatric sepsis biomarker risk model. PLoS One. 2014. 9(1):e86242. [Medline]. [Full Text].
[Guideline] de Oliveira CF, de Oliveira DS, Gottschald AF, Moura JD, Costa GA, Ventura AC, et al. ACCM/PALS haemodynamic support guidelines for paediatric septic shock: an outcomes comparison with and without monitoring central venous oxygen saturation. Intensive Care Med. 2008 Jun. 34(6):1065-75. [Medline].
Sankar J, Sankar MJ, Suresh CP, Dubey NK, Singh A. Early goal-directed therapy in pediatric septic shock: comparison of outcomes "with" and "without" intermittent superior venacaval oxygen saturation monitoring: a prospective cohort study*. Pediatr Crit Care Med. 2014 May. 15(4):e157-67. [Medline].
Dias CR, Leite HP, Nogueira PC, Brunow de Carvalho W. Ionized hypocalcemia is an early event and is associated with organ dysfunction in children admitted to the intensive care unit. J Crit Care. 2013 Oct. 28(5):810-5. [Medline].
Broner CW, Stidham GL, Westenkirchner DF, Watson DC. A prospective, randomized, double-blind comparison of calcium chloride and calcium gluconate therapies for hypocalcemia in critically ill children. J Pediatr. 1990 Dec. 117(6):986-9. [Medline].
Meert KL, Donaldson A, Nadkarni V, Tieves KS, Schleien CL, Brilli RJ, et al. Multicenter cohort study of in-hospital pediatric cardiac arrest. Pediatr Crit Care Med. 2009 Sep. 10(5):544-53. [Medline]. [Full Text].
Carcillo JA, Davis AL, Zaritsky A. Role of early fluid resuscitation in pediatric septic shock. JAMA. 1991 Sep 4. 266(9):1242-5. [Medline].
Ranjit S, Kissoon N, Jayakumar I. Aggressive management of dengue shock syndrome may decrease mortality rate: a suggested protocol. Pediatr Crit Care Med. 2005 Jul. 6(4):412-9. [Medline].
Oliveira CF, Nogueira de Sá FR, Oliveira DS, Gottschald AF, Moura JD, Shibata AR, et al. Time- and fluid-sensitive resuscitation for hemodynamic support of children in septic shock: barriers to the implementation of the American College of Critical Care Medicine/Pediatric Advanced Life Support Guidelines in a pediatric intensive care unit in a developing world. Pediatr Emerg Care. 2008 Dec. 24(12):810-5. [Medline].
Voigt J, Waltzman M, Lottenberg L. Intraosseous vascular access for in-hospital emergency use: a systematic clinical review of the literature and analysis. Pediatr Emerg Care. 2012 Feb. 28(2):185-99. [Medline].
Cole ET, Harvey G, Urbanski S, Foster G, Thabane L, Parker MJ. Rapid paediatric fluid resuscitation: a randomised controlled trial comparing the efficiency of two provider-endorsed manual paediatric fluid resuscitation techniques in a simulated setting. BMJ Open. 2014 Jul 3. 4(7):e005028. [Medline]. [Full Text].
Maitland K, Kiguli S, Opoka RO, Engoru C, Olupot-Olupot P, Akech SO, et al. Mortality after fluid bolus in African children with severe infection. N Engl J Med. 2011 Jun 30. 364(26):2483-95. [Medline].
Abulebda K, Cvijanovich NZ, Thomas NJ, Allen GL, Anas N, Bigham MT, et al. Post-ICU admission fluid balance and pediatric septic shock outcomes: a risk-stratified analysis. Crit Care Med. 2014 Feb. 42(2):397-403. [Medline]. [Full Text].
Weiss SL, Fitzgerald JC, Balamuth F, Alpern ER, Lavelle J, Chilutti M, et al. Delayed antimicrobial therapy increases mortality and organ dysfunction duration in pediatric sepsis. Crit Care Med. 2014 Nov. 42(11):2409-17. [Medline]. [Full Text].
[Guideline] Kleinman ME, Chameides L, Schexnayder SM, Samson RA, Hazinski MF, Atkins DL, et al. Pediatric advanced life support: 2010 American Heart Association Guidelines for Cardiopulmonary Resuscitation and Emergency Cardiovascular Care. Pediatrics. 2010 Nov. 126(5):e1361-99. [Medline].
Hanna W, Wong HR. Pediatric sepsis: challenges and adjunctive therapies. Crit Care Clin. 2013 Apr. 29(2):203-22. [Medline]. [Full Text].
Alejandria MM, Lansang MA, Dans LF, Mantaring JB 3rd. Intravenous immunoglobulin for treating sepsis, severe sepsis and septic shock. Cochrane Database Syst Rev. 2013 Sep 16. 9:CD001090. [Medline].
Kissoon N, Carcillo JA, Espinosa V, Argent A, Devictor D, Madden M, et al. World Federation of Pediatric Intensive Care and Critical Care Societies: Global Sepsis Initiative. Pediatr Crit Care Med. 2011 Sep. 12(5):494-503. [Medline].
Zimmerman JJ, Williams MD. Adjunctive corticosteroid therapy in pediatric severe sepsis: observations from the RESOLVE study. Pediatr Crit Care Med. 2011 Jan. 12(1):2-8. [Medline].
Atkinson SJ, Cvijanovich NZ, Thomas NJ, Allen GL, Anas N, Bigham MT, et al. Corticosteroids and pediatric septic shock outcomes: a risk stratified analysis. PLoS One. 2014. 9(11):e112702. [Medline]. [Full Text].
Menon K, McNally D, Choong K, Sampson M. A systematic review and meta-analysis on the effect of steroids in pediatric shock. Pediatr Crit Care Med. 2013 Jun. 14(5):474-80. [Medline].
Pizarro CF, Troster EJ, Damiani D, Carcillo JA. Absolute and relative adrenal insufficiency in children with septic shock. Crit Care Med. 2005 Apr. 33(4):855-9. [Medline].
Schotola H, Toischer K, Popov AF, Renner A, Schmitto JD, Gummert J, et al. Mild metabolic acidosis impairs the ß-adrenergic response in isolated human failing myocardium. Crit Care. 2012 Aug 13. 16(4):R153. [Medline]. [Full Text].
Mathieu D, Neviere R, Billard V, Fleyfel M, Wattel F. Effects of bicarbonate therapy on hemodynamics and tissue oxygenation in patients with lactic acidosis: a prospective, controlled clinical study. Crit Care Med. 1991 Nov. 19(11):1352-6. [Medline].
Aschner JL, Poland RL. Sodium bicarbonate: basically useless therapy. Pediatrics. 2008 Oct. 122(4):831-5. [Medline].
Paden ML, Rycus PT, Thiagarajan RR. Update and outcomes in extracorporeal life support. Semin Perinatol. 2014 Mar. 38(2):65-70. [Medline].
DiCarlo JV, Dudley TE, Sherbotie JR, Kaplan BS, Costarino AT. Continuous arteriovenous hemofiltration/dialysis improves pulmonary gas exchange in children with multiple organ system failure. Crit Care Med. 1990 Aug. 18(8):822-6. [Medline].
Foland JA, Fortenberry JD, Warshaw BL, Pettignano R, Merritt RK, Heard ML, et al. Fluid overload before continuous hemofiltration and survival in critically ill children: a retrospective analysis. Crit Care Med. 2004 Aug. 32(8):1771-6. [Medline].
Vogt W, Laer S. Prevention for pediatric low cardiac output syndrome: results from the European survey EuLoCOS-Paed. Paediatr Anaesth. 2011 Dec. 21(12):1176-84. [Medline].
Hoffman TM. Newer inotropes in pediatric heart failure. J Cardiovasc Pharmacol. 2011 Aug. 58(2):121-5. [Medline].
Hoffman TM, Wernovsky G, Atz AM, Kulik TJ, Nelson DP, Chang AC, et al. Efficacy and safety of milrinone in preventing low cardiac output syndrome in infants and children after corrective surgery for congenital heart disease. Circulation. 2003 Feb 25. 107(7):996-1002. [Medline].
Meyer S, Gortner L, Brown K, Abdul-Khaliq H. The role of milrinone in children with cardiovascular compromise: review of the literature. Wien Med Wochenschr. 2011 Apr. 161(7-8):184-91. [Medline].
Bassler D, Kreutzer K, McNamara P, Kirpalani H. Milrinone for persistent pulmonary hypertension of the newborn. Cochrane Database Syst Rev. 2010 Nov 10. CD007802. [Medline].