Coronavirus Disease 2019 (COVID-19)
- Various
- 27 jun 2023
- 87 Min. de lectura
Updated: Apr 06, 2023
Overview
Practice Essentials
Coronavirus disease 2019 (COVID-19) is defined as illness caused by a novel coronavirus called severe acute respiratory syndrome coronavirus 2 (SARS-CoV-2; formerly called 2019-nCoV), which was first identified amid an outbreak of respiratory illness cases in Wuhan City, Hubei Province, China. [1] It was initially reported to the WHO on December 31, 2019. On January 30, 2020, the WHO declared the COVID-19 outbreak a global health emergency. [2, 3] On March 11, 2020, the WHO declared COVID-19 a global pandemic, its first such designation since declaring H1N1 influenza a pandemic in 2009. [4]
Illness caused by SARS-CoV-2 was termed COVID-19 by the WHO, the acronym derived from "coronavirus disease 2019." The name was chosen to avoid stigmatizing the virus's origins in terms of populations, geography, or animal associations. [5, 6] On February 11, 2020, the Coronavirus Study Group of the International Committee on Taxonomy of Viruses issued a statement announcing an official designation for the novel virus: severe acute respiratory syndrome coronavirus 2 (SARS-CoV-2). [7]
The CDC estimates that SARS-CoV-2 entered the United States in late January or early February 2020, establishing low-level community spread before being noticed. [8] Since that time, the United States has experienced widespread infections, with over 97.6 million reported cases and over 1,125,000 deaths reported as of March 29, 2023 as reported by the CDC COVID data tracker. According to the CDC, 75% of people who have died of the virus in the United States as of April 5, 2023 are aged 65 years or older. According to the New York Times, the CDC reports that 1 in 100 older Americans has died from the virus. For people younger than 65, the ratio is about 1 in 1,400.
On April 3, 2020, the CDC issued a recommendation that the general public, even those without symptoms, should begin wearing face coverings in public settings where social-distancing measures are difficult to maintain to abate the spread of COVID-19. [9]
The CDC had postulated that large numbers of patients could require medical care concurrently, resulting in overloaded public health and healthcare systems and, potentially, elevated rates of hospitalizations and deaths. The CDC advised that nonpharmaceutical interventions (NPIs) are the most important response strategy for delaying viral spread and reducing disease impact. Unfortunately, these concerns have been proven accurate.
The feasibility and implications of suppression and mitigation strategies have been rigorously analyzed and are being encouraged or enforced by many governments to slow or halt viral transmission. Population-wide social distancing plus other interventions (eg, home self-isolation, school and business closures) are strongly advised. These policies may be required for long periods to avoid rebound viral transmission. [10]
As the United States is experiencing another surge of COVID-19 infections, the CDC has intensified its recommendations for transmission mitigation. They recommend all unvaccinated individuals wear masks in public indoor settings. On the basis of evidence regarding emerging variants of concern (See Virology), CDC recommends that persons who are fully vaccinated also wear masks in public indoor settings in areas with substantial or high transmission. Fully vaccinated individuals might consider wearing a mask in public indoor areas, regardless of transmission level, if they or someone in their home is immunocompromised, is at increased risk for severe disease, or is unvaccinated (including children younger than 12 years who are ineligible for vaccination). [11]
The CDC recommends physical distancing, avoiding nonessential indoor spaces, postponing travel until fully vaccinated, enhanced ventilation, and hand hygiene. [12, 13]
According to the CDC, individuals at high risk for infection include persons in areas with ongoing local transmission, healthcare workers caring for patients with COVID-19, close contacts of infected persons, and travelers returning from locations where local spread has been reported.
The CDC has published a summary of evidence of comorbidities that are supported by meta-analysis/systematic review that have a significant association with risk of severe COVID-19 illness. These include the following conditions [14] :
Cancer
Cerebrovascular disease
Chronic kidney disease
COPD (chronic obstructive pulmonary disease)
Diabetes mellitus, type 1 and type 2
Heart conditions (eg, heart failure, coronary artery disease, cardiomyopathies)
Immunocompromised state from solid organ transplant
Obesity (BMI 30 kg/m 2 or greater)
Pregnancy
Smoking, current or former
Comorbidities that are supported by mostly observational (eg, cohort, case-control, or cross-sectional) studies include the following [14] :
Children with certain underlying conditions
Down syndrome
HIV (human immunodeficiency virus)
Neurologic conditions, including dementia
Overweight (BMI 25 to less than 30 kg/m 2)
Other lung disease (including interstitial lung disease, pulmonary fibrosis, pulmonary hypertension)
Sickle cell disease
Solid organ or blood stem cell transplantation
Substance use disorders
Use of corticosteroids or other immunosuppressive medications
Comorbidities that are supported by mostly case series, case reports, or, if other study design or the sample size is small include the following [14] :
Cystic fibrosis
Thalassemia
Comorbidities supported by mixed evidence include the following [14] :
Asthma
Hypertension
Immune deficiencies
Liver disease
Such individuals should consider the following precautions [14] :
Stock up on supplies.
Avoid close contact with sick people.
Wash hands often.
Stay home as much as possible in locations where COVID-19 is spreading.
Develop a plan in case of illness.
Signs and symptoms
In a study that included 172 patients diagnosed with COVID-19 in January 2022, the estimated median incubation period was 2.8 days (SD, 1.20) among those infected with the Omicron variant (primarily sublineage BA.1). Most infections fell between 1 and 6 days. The distribution was significantly longer in patients with the Alpha variant (4.5 days), and the researchers’ previous study that used contact tracing data estimated a median incubation period of 3.7 days for the Delta variant. [15]
The following symptoms may indicate COVID-19 [16] :
Fever or chills (43-45%)
Cough (63-83%)
Shortness of breath or difficulty breathing (45.6%) [17]
Fatigue (63%)
Muscle or body aches (36-63%)
Headache (34-70%)
New loss of taste (54.2%) or smell (70.2%)
Sore throat (52.9%)
Congestion (67.8%) or runny nose (60.1%)
Nausea or vomiting (31.6%) [18]
Diarrhea (17.8%) [18]
Other reported symptoms have included the following:
Sputum production
Malaise
Respiratory distress
Neurologic (eg, headache, altered mentality)
The most common serious manifestation of COVID-19 appears to be pneumonia.
A complete or partial loss of the sense of smell (anosmia) has been reported as a potential history finding in patients eventually diagnosed with COVID-19 [19] ; however, rates of smell or taste dysruption have decreased as the pandemic has progressed. A study of 616,318 patients with COVID-19 found that 3431 had an associated disturbance in smell or taste; of those, the odds ratios were 0.50 among those infected with the Alpha variant; 0.44 among those infected with Delta; and 0.17 among those infected with Omicron (December 27, 2021–February 7, 2022). [20]
Diagnosis
COVID-19 should be considered a possibility (1) in patients with respiratory tract symptoms and newly onset fever or (2) in patients with severe lower respiratory tract symptoms with no clear cause. Suspicion is increased if such patients have been in an area with community transmission of SARS-CoV-2 or have been in close contact with an individual with confirmed or suspected COVID-19 in the preceding 14 days.
Microbiologic (PCR or antigen) testing is required for definitive diagnosis.
Patients who do not require emergency care are encouraged to contact their healthcare provider by phone. Patients with suspected COVID-19 who present to a healthcare facility should trigger infection-control measures. These patients should be evaluated in a private room with the door closed (an airborne infection isolation room is ideal) and instructed to wear a surgical mask. All other standard contact and airborne precautions should be observed, and treating healthcare personnel should wear eye protection. [21]
Management
Utilization of programs established by the FDA to allow clinicians access to investigational therapies during the pandemic has been essential. The expanded access (EA) and emergency use authorization (EUA) programs allowed for rapid deployment of potential therapies for investigation and investigational therapies with emerging evidence. A review by Rizk et al describes the role for each of these measures and their importance to providing medical countermeasures in the event of infectious disease and other threats. [22]
Remdesivir, an antiviral agent, was the first drug to gain full FDA approval for treatment of hospitalized adults and adolescents with COVID-19 disease in October 2020. [23] Since then, it has gained approval for adults and pediatric patients (aged 28 days and older who weigh at least 3 kg) with positive results of direct SARS-CoV-2 viral testing, who are hospitalized, or not hospitalized and have mild-to-moderate COVID-19, and are at high risk for progression to severe COVID-19, including hospitalization or death. [24]
The first vaccine to gain full FDA approval was mRNA-COVID-19 vaccine (Comirnaty; Pfizer) in August 2021. A second mRNA vaccine (Spikevax; Moderna) was approved by the FDA in January 2022. Additionally, each of these vaccines have EUAs for children as young as 6 months.EUAs have been issued for other vaccines.
Baricitinib (Olumiant), a Janus kinase inhibitor, gained FDA approval for hospitalized adults with COVID-19 disease who require supplemental oxygen, noninvasive or invasive mechanical ventilation, or ECMO. An EUA for children has been issued for baricitinib.
Similar to baricitinib, tocilizumab (Actemra), an interleukin 6 inhibitor, was approved by the FDA for hospitalized adults. An EUA remains in place for children aged 2 years and older.
EUAs have also been issued for other antivirals, vaccines and convalescent plasma in the United States. A full list of EUAs and access to the Fact Sheets for Healthcare Providers is available from the FDA.
Use of corticosteroids improves survival in hospitalized patients with severe COVID-19 disease requiring supplemental oxygen, with the greatest benefit shown in those requiring mechanical ventilation. [25]
Infected patients should receive supportive care to help alleviate symptoms. Vital organ function should be supported in severe cases. [26]
Numerous collaborative efforts to discover and evaluate effectiveness of antivirals, immunotherapies, monoclonal antibodies, and vaccines have rapidly emerged. Guidelines and reviews of pharmacotherapy for COVID-19 have been published. [26, 27, 28, 29, 30]
Background
Coronaviruses comprise a vast family of viruses, seven of which are known to cause disease in humans. Some coronaviruses that typically infect animals have evolved to infect humans. SARS-CoV-2 is likely one such virus, postulated to have originated in a large animal and seafood market.
Severe acute respiratory syndrome (SARS) and Middle East respiratory syndrome (MERS) also are caused by coronaviruses that “jumped” from animals to humans. More than 8000 individuals developed SARS, nearly 800 of whom died of the illness (mortality rate, approximately 10%), before it was controlled in 2003. [31] MERS continues to resurface in sporadic cases. In late February 2022, a total of 2585 laboratory-confirmed cases of Middle East respiratory syndrome (MERS) were reported worldwide (890 associated deaths; case-fatality ratio, 34.4%). [32]
Route of Transmission
The principal mode by which people are infected with SARS-CoV-2 is through exposure to respiratory droplets carrying infectious virus (generally within a space of 6 feet). Additional methods include contact transmission (eg, shaking hands) and airborne transmission [33] of droplets that linger in the air over long distances (usually greater than 6 feet). [34, 35, 36] Virus released in respiratory secretions (eg, during coughing, sneezing, talking) can infect other individuals via contact with mucous membranes.
On July 9, 2020, the WHO issued an update stating that airborne transmission may play a role in the spread of COVID-19, particularly involving “super spreader” events in confined spaces such as bars, although they stressed a lack of such evidence in medical settings. Thus, they emphasized the importance of social distancing and masks in prevention. [37] The WHO continues to support airborne transmission as a method of the disease's spread. [38]
The virus can also persist on surfaces to varying durations and degrees of infectivity, although this is not believed to be the main route of transmission. [35] One study found that SARS-CoV-2 remained detectable for up to 72 hours on some surfaces despite decreasing infectivity over time. Notably, the study reported that no viable SARS-CoV-2 was measured after 4 hours on copper or after 24 hours on cardboard. [39]
In a separate study, Chin and colleagues found the virus was very susceptible to high heat (70°C). At room temperature and moderate (65%) humidity, no infectious virus could be recovered from printing and tissue papers after a 3-hour incubation period or from wood and cloth by day 2. On treated smooth surfaces, infectious virus became undetectable from glass by day 4 and from stainless steel and plastic by day 7. “Strikingly, a detectable level of infectious virus could still be present on the outer layer of a surgical mask on day 7 (~0.1% of the original inoculum),” the researchers write. [40] Contact with fomites is thought to be less significant than person-to-person spread as a means of transmission. [35]
Viral shedding
The duration of viral shedding varies significantly and may depend on severity. A 2022 sytematic review and meta-analysis of 20 studies (866 participants) found that after symptom onset, the mean duration of RT-PCR positivity was 27.9 days, whereas the mean duration of isolation of replicant competent virus was 7.3 days. The mean duration of SARS-CoV-2 shedding was 26.5 days among immunocompetent individuals and 36.3 days among immunocompromised individuals. The mean duration of infectivity was 6.3 days among immunocompetent participants and 29.5 days among immunocompromised participants. The longest duration of infectivity was 18 days after symptom onset among immunocompetent patients compared with a maximum of 112 days among immunocompromised patients. [41]
Among 137 survivors of COVID-19, viral shedding based on testing of oropharyngeal samples ranged from 8 to 37 days, with a median of 20 days. [42] A different study found that repeated viral RNA tests using nasopharyngeal swabs were negative in 90% of cases among 21 patients with mild illness, whereas results were positive for longer durations in patients with severe COVID-19. [43] In an evaluation of patients recovering from severe COVID-19, Zhou and colleagues found a median shedding duration of 31 days (range, 18-48 days). [44] These studies have all used PCR detection as a proxy for viral shedding. The Korean CDC, investigating a cohort of patients who had prolonged PCR positivity, determined that infectious virus was not present. [45] These findings were incorporated into the CDC guidance on the duration of isolation following COVID-19 infection.
Additionally, patients with profound immunosuppression (eg, following hematopoietic stem-cell transplantation, receiving cellular therapies) may shed viable SARS-CoV-2 for at least 2 months. [46, 47]
SARS-CoV-2 has been found in the semen of men with acute infection, as well as in some male patients who have recovered. [48]
Asymptomatic/presymptomatic SARS-CoV-2 infection and its role in transmission
Oran and Topol published a narrative review of multiple studies on asymptomatic SARS-CoV-2 infection. Such studies and news articles reported rates of asymptomatic infection in several worldwide cohorts, including resident populations from Iceland and Italy, passengers and crew aboard the cruise ship Diamond Princess, homeless persons in Boston and Los Angeles, obstetric patients in New York City, and crew aboard the USS Theodore Roosevelt and Charles de Gaulle aircraft carriers, among several others. Almost half (40-45%) of SARS-CoV-2 infections were asymptomatic. [49]
Johansson et al from the CDC assessed transmission from presymptomatic, never symptomatic, and symptomatic individuals across various scenarios to determine the infectious period of transmitting SARS-CoV-2. Results from their base case determined 59% of all transmission came from asymptomatic transmission, 35% from presymptomatic individuals and 24% from individuals who never developed symptoms. They estimate at least 50% of new infections came from exposure to individuals with infection, but without symptoms. [50]
Zou and colleagues followed viral expression through infection via nasal and throat swabs in a small cohort of patients. They found increases in viral loads at the time that the patients became symptomatic. One patient never developed symptoms but was shedding virus beginning at day 7 after presumed infection. [51]
Epidemiology
Coronavirus outbreak and pandemic
As of April 5, 2023, confirmed COVID-19 infections numbered over 762 million individuals worldwide and have resulted in nearly 7 million deaths. [52] Additionally, the World Health Organization estimates the full death toll associated directly or indirectly with the pandemic is approximately 15 million.
In the United States, over 104 million reported cases of COVID-19 have been confirmed as of March 29, 2023, resulting in more than 1,125,000 deaths. [53] The pandemic caused approximately 375,000 deaths in the United States during 2020. The age-adjusted death rate increased by 15.9% in 2020, making it the third leading cause of death after heart disease and cancer. [54] During 2021, COVID-19 was associated with 416,893 deaths in the United States, and was again the third leading cause of death. Approximately 75% of deaths in the United States from COVID-19 occurred in individuals aged 65 years and older. [55]
Racial health disparities
Communities of color have been disproportionally devastated by COVID-19 in the United States and in Europe. Data from New Orleans illustrated these disparities. African Americans represent 31% of the population but 76.9% of the hospitalizations and 70.8% of the deaths. [56]
A systematic review of 52 studies found racial and ethnic minority groups were at higher risk for COVID-19 infection and hospitalization, confirmed diagnosis, and death. Most of the studies listed factors such as low education level, poverty, poor housing conditions and overcrowded households, low household income, and not speaking the national language in a country as risk factors for COVID-19 incidence/infection, death, and confirmed diagnosis. [57]
Data suggest the cumulative effects of health disparities are the driving force. The prevalence of chronic (high-risk) medical conditions is higher, and access to healthcare may be less available. Finally, socioeconomic status may decrease the ability to isolate and avoid infection. [58, 59]
A prospective cohort study surveyed 170 adult patients who had recovered from COVID-19 1 year prior, during March and April of 2020. The patients participated in a telephone survey during March and April of 2021.
Almost half (79 patients; 46.5%) were of Hispanic ethnicity and 27.1% (46 patients) were African American. Job loss after COVID-19 diagnosis was highest among Hispanics (31/79; 39.2%) and African Americans (16/46; 34.7%). Hispanic individuals (31/79; 39.2%) and African Americans (17/46; 36.9%) also reported the most financial distress after COVID-19 diagnosis.
Compared with Whites, Hispanics were more likely to experience job loss (odds ratio [OR], 4.456), as were African Americans (OR, 4.465). [60]
Hobbs et al compared MIS-C cases (38 cases) and COVID-19 hospitalizations (74 children) among non-Hispanic Black and White children in a defined catchment 16-county area of Mississippi.
Compared with White children, Black children had an almost fivefold cumulative incidence of MIS-C (40.7 vs 8.3 cases per 100,000 SARS-CoV-2 infections). The cumulative incidence of hospitalization for COVID-19 was almost twice as high in Black children compared with White children (62.3 among Black vs 33.1 among White children per 100,000 SARS-CoV-2 infections). [61]
Ward et al conducted a retrospective analysis of COVID-19 cases reported to the Alaska Department of Health and Social Services from March 12, 2020-December 31, 2021. The age-adjusted COVID-19 incidence among American Indian (AI)/Alaska Native (AN) individuals (26,583 per 100,000 standard population) was approximately twice the rate among White individuals (11,935).
The age-adjusted COVID-19-associated hospitalization rate (273; rate ratio [RR], 2.72) and the age-adjusted COVID-19 related mortality rate (104; RR, 2.86) among AI/AN individuals were nearly three times those of White study participants. [62]
The overall age-adjusted death rate increased by 15.9% in 2020. Death rates were highest among non-Hispanic Black persons and non-Hispanic American Indian or Alaska Native persons. [54]
CDC maintains a COVID-19 Data Tracker for near real time updates.
Young Adults
Outcomes from COVID-19 disease in young adults have been described by Cunningham and colleagues. Of 3200 adults aged 18 to 34 years hospitalized in the United States with COVID-19, 21% were admitted to the ICU, 10% required mechanical ventilation, and 3% died. Comorbidities included obesity (33%; 25% overall were morbidly obese), diabetes (18%), and hypertension (16%). Independent predictors of death or mechanical ventilation included hypertension, male sex, and morbid obesity. Young adults with multiple risk factors for poor outcomes from COVID-19 compared similarly to middle-aged adults without such risk factors. [63]
A study from South Korea found that older children and adolescents are more likely to transmit SARS CoV-19 to family members than are younger children. The researchers reported that the highest infection rate (18.6%) was in household contacts of patients with COVID-19 aged 10 to 19 years, and the lowest rate (5.3%) was in household contacts of those aged 0 to 9 years. [64] Teenagers have been the source of clusters of cases, illustrating the role of older children. [65]
COVID-19 in children
Data continue to emerge regarding the incidence and effects of COVID-19, especially for severe disease. [66] A severe multisystem inflammatory syndrome linked to COVID-19 infection has been described in children. [67, 68, 69, 70, 66]
The American Academy of Pediatrics (AAP) reports children represent 18.3% of all COVID-19 cases in the 49 states reporting by age; nearly 15 million children have tested positive in the United States since the onset of the pandemic as of October 27, 2022. This represents an overall rate of 19,787 cases per 100,000 children. During the 2-week period of October13-27, 2022, there was less than a 1% increase in the cumulated number of children who tested positive, representing 47,261 new confirmed cases. In the week from October 20-27, 2022, cases in children numbered 14,868 and represented 11.1% of the new weekly cases. [71]
AAP has issued interim guidance for follow-up care of children following a SARS-CoV-2 infection.
In the United States, a modeling study found one child loses a parent or caregiver for every four COVID-19 associated deaths. From April 1, 2020 through June 30, 2021, more than 140,000 children younger than 18 years in the United States lost a parent, custodial grandparent, or grandparent caregiver who provided the child’s home and basic needs, including love, security, and daily care. Overall, approximately one of 500 US children has experienced COVID-19-associated orphanhood or the death of a grandparent caregiver. Racial, ethnic, and geographic disparities in COVID-19-associated death of caregivers were also seen – children of racial and ethnic minorities accounted for 65% of those who lost a primary caregiver due to the pandemic. [72]
As of late June 2022, approximately 85,825,048 cases of SARS-CoV-2 infection and 1,007,964 associated deaths have been reported in the United States. [73] Persons younger than 21 years constitute 26% of the US population. [74]
Clinical characteristics and outcomes of hospitalized children and adolescents aged 1 month to 21 years with COVID-19 in the New York City area have been described. These observations alerted clinicians to rare, but severe illness in children. Of 67 children who tested positive for COVID-19, 21 (31.3%) were managed as outpatients. Among 46 hospitalized patients, 33 (72%) were admitted to the general pediatric medical unit and 13 (28%) to the pediatric intensive care unit (PICU). Obesity and asthma were highly prevalent, but not significantly associated with PICU admission (P = .99).
Admission to the pediatric intensive care unit (PICU) was significantly associated with higher C-reactive protein, procalcitonin, and pro-B type natriuretic peptide levels and platelet counts (P < .05 for all). Patients in the PICU were more likely to require high-flow nasal cannula (P = .0001) and were more likely to have received remdesivir through compassionate release (P < .05). Severe sepsis and septic shock syndromes were observed in 7 (53.8%) patients in the PICU. ARDS was observed in 10 (77%) PICU patients, 6 (46.2%) of whom required invasive mechanical ventilation for a median of 9 days. Of the 13 patients in the PICU, 8 (61.5%) were discharged home, and 4 (30.7%) patients remained hospitalized on ventilatory support at Day 14. One patient died after withdrawal of life-sustaining therapy associated with metastatic cancer. [75]
A case series of 91 children who tested positive for COVID-19 in South Korea showed 22% were asymptomatic during the entire observation period. Among 71 symptomatic cases, 47 children (66%) had unrecognized symptoms before diagnosis, 18 (25%) developed symptoms after diagnosis, and 6 (9%) were diagnosed at the time of symptom onset. Twenty-two children (24%) had lower respiratory tract infections. The mean (SD) duration of the presence of SARS-CoV-2 RNA in upper respiratory samples was 17.6 (6.7) days. These results lend more data to unapparent infections in children that may be associated with silent COVID-19 community transmission. [76]
An Expert Consensus Statement has been published that discusses diagnosis, treatment, and prevention of COVID-19 in children.
Multisystem inflammatory syndrome in children
Media reports and a health alert from the New York State Department of Health drew initial attention to a newly recognized multisystem inflammatory syndrome in children (MIS-C) associated with COVID-19. Since then, MIS-C cases have been reported across the United States and Europe, and the American Academy of Pediatrics has published interim guidance.
Symptoms are reminiscent of Kawasaki disease, atypical Kawasaki disease, or toxic shock syndrome. All patients had persistent fevers, and more than half had rashes and abdominal complaints. Interestingly, respiratory symptoms were rarely described. Many patients did not have PCR results positive for COVID-19, but many had strong epidemiologic links with close contacts who tested positive. Furthermore, many had antibody tests positive for SARS-CoV-2. These findings suggest recent past infection, and this syndrome may be a postinfectious inflammatory syndrome. The CDC case definition requires:
An individual younger than 21 years presenting with fever ≥38.0°C for ≥24 hours, laboratory evidence of inflammation (including an elevated C-reactive protein [CRP], erythrocyte sedimentation rate [ESR], fibrinogen, procalcitonin, D-dimer, ferritin, lactic acid dehydrogenase [LDH], or interleukin 6 [IL-6], elevated neutrophils, reduced lymphocytes, and low albumin), and evidence of clinically severe illness requiring hospitalization, with multisystem (≥2) organ involvement (cardiac, renal, respiratory, hematologic, gastrointestinal, dermatologic, or neurological); AND
No alternative plausible diagnoses; AND
Positive for current or recent SARS-CoV-2 infection by RT-PCR, serology, or antigen test; or exposure to a suspected or confirmed COVID-19 case within the 4 weeks prior to the onset of symptoms.
Jiang and colleagues reviewed the literature on MIS-C noting the multiple organ system involvement. Unlike classic Kawasaki Disease, the children tended to be older and those of Asian ethnicity tended to be spared. [77]
A case series compared 539 patients who had MIS-C with 577 children and adolescents who had severe COVID-19. The patients with MIS-C were typically younger (predominantly aged 6-12 years) and more likely to be non-Hispanic Black. They were less likely to have an underlying chronic medical condition, such as obesity. Severe cardiovascular or mucocutaneous involvement was more common in those with MIS-C. Patients with MIS-C also had higher neutrophil to lymphocyte ratios, higher CRP levels, and lower platelet counts than those with severe COVID-19. [78]
COVID-19 in pregnant individuals and neonates
Pregnant women are at increased risk for severe COVID-19–related illness, and COVID-19 is associated with an increased risk for adverse pregnancy outcomes including intrauterine growth restriction, premature rupture of membranes and preterm delivery, fetal distress, spontaneous abortion, and stillbirth, and maternal and neonatal complications. [79, 80, 81]
The Centers for Disease Control and Prevention reported in November 2021 that maternal COVID-19 infection increases risk for stillbirth compared with women without COVID-19. From March 2020 to September 2021, 8154 stillbirths were reported, affecting 0.65% of births by women without COVID and 1.26% of births by women with COVID, for a relative risk (RR) of 1.90. The magnitude of association was higher during the period of SARS-CoV-2 B.1.617.2 (Delta) variant predominance than during the pre-Delta period. [82]
A multicenter study involving 16 Spanish hospitals reported outcomes of 242 pregnant patients diagnosed with COVID-19 during the third trimester from March 13 to May 31, 2020. They and their 248 newborns were monitored until the infant was 1 month old. Pregnant patients with COVID-19 who were hospitalized had a higher risk for cesarean birth (P = 0.027). Newborns whose mothers were hospitalized for COVID-19 infection had a higher risk for premature delivery (P = 0.006). No infants died and no vertical or horizontal transmission was detected. Exclusive breastfeeding was reported for 41.7% of infants at discharge and 40.4% at 1 month. [83]
A cohort study of pregnant patients (n = 64) with severe or critical COVID-19 disease hospitalized at 12 US institutions between March 5, 2020, and April 20, 2020 has been published. At the time of the study, most (81%) received hydroxychloroquine; 7% of those with severe disease and 65% with critical disease received remdesivir. All of those with critical disease received either prophylactic or therapeutic anticoagulation. One case of maternal cardiac arrest occurred, but there were no cases of cardiomyopathy or death. Half (n = 32) delivered during their hospitalization (34% severe group; 85% critical group). Additionally, 88% with critical disease delivered preterm during their disease course, with 16 of 17 (94%) pregnant women giving birth through cesarean delivery. Overall, 15 of 20 (75%) with critical disease delivered preterm. There were no stillbirths or neonatal deaths or cases of vertical transmission. [84]
Adhikari and colleagues published a cohort study evaluating 252 pregnant patients with COVID-19 in Texas. Maternal illness at initial presentation was asymptomatic or mild in 95% of them, and 3% developed severe or critical illness. Compared with COVID negative pregnancies, there was no difference in the composite primary outcome of preterm birth, preeclampsia with severe features, or cesarean delivery for abnormal fetal heart rate. Early neonatal SARS-CoV-2 infection occurred in six of 188 tested infants,(3%) primarily born to asymptomatic or mildly symptomatic mothers. There were no placental pathologic differences by illness severity. [85]
Breastfeeding
A study by Chambers and colleagues found human milk is unlikely to transmit SARS-CoV-2 from infected mothers to infants. The study included 64 milk samples provided by 18 mothers infected with COVID-19. Samples were collected before and after COVID-19 diagnosis. No replication-competent virus was detectable in any of their milk samples compared with samples of human milk that were experimentally infected with SARS-CoV-2. [86]
Mothers or birthing parents who have been infected with SARS CoV-2 may have neutralizing antibodies expressed in their milk. In an evaluation of 1-7 milk samples over 2 months from 64 women, 75% contained SARS-CoV-2-specific IgA and 7% persited for at least 2 months. These results support recommendations to continue breastfeeding/chestfeeding with masking during mild-to-moderate maternal COVID-19 illness. [87]
COVID-19 in patients with HIV
Data for people with HIV and coronavirus are emerging. A multicenter registry has published outcomes for 286 patients with HIV who tested positive for COVID-19 between April 1 and July 1, 2020. Patient characteristics included mean age of 51.4 years, 25.9% were female, and 75.4% were African-American or Hispanic. Most patients (94.3%) were on antiretroviral therapy, 88.7% had HIV virologic suppression, and 80.8% had comorbidities. Within 30 days of positive SARS-CoV-2 testing, 164 (57.3%) patients were hospitalized, and 47 (16.5%) required ICU admission. Mortality rates were 9.4% (27/286) overall, 16.5% (27/164) among those hospitalized, and 51.5% (24/47) among those admitted to an ICU. [88]
Multiple case series have subsequently been published. Most suggest similar outcomes in patients living with HIV as the general patient population. [89, 90] Severe COVID-19 has been seen, however, suggesting that neither antiretroviral therapy of HIV infection are protective. [88, 91]
A systematic review and meta-analysis of 43 studies including 692,032 COVID-19 cases found that 9097 (1.3%) were among people living with HIV (PLWH); the global prevalence of PLWH among cases of COVID-19 was 2%, and the highest prevalence occurred in sub-Saharan Africa. The relative risk (RR) for severe COVID-19 in PLWH was significant only in Africa, at 1.14, whereas the RR for mortality was 1.5 worldwide, suggesting that HIV infection may be associated with increased death from COVID-19. [92]
COVID-19 in clinicians
Among a sample of healthcare providers who routinely cared for patients with COVID-19 in 13 US academic medical centers from February 1, 2020, 6% had evidence of previous SARS-CoV-2 infection, with considerable variation by location that generally correlated with community cumulative incidence. Among participants who had positive test results for SARS-CoV-2 antibodies, approximately one third did not recall any symptoms consistent with an acute viral illness in the preceding months, nearly one half did not suspect that they previously had COVID-19, and approximately two thirds did not have a previous positive test result demonstrating an acute SARS-CoV-2 infection. [93]
Prognosis
During January to December 2020, the estimated 2020 age-adjusted death rate increased for the first time since 2017, with an increase of 15.9% compared with 2019, from 715.2 to 828.7 deaths per 100,000 population. COVID-19 was the underlying or a contributing cause of 377,883 deaths (91.5 deaths per 100,000). COVID-19 death rates were highest among males, older adults, non-Hispanic American Indian or Alaska Native (AI/AN) persons, and Hispanic persons. Age-adjusted death rates was highest among Black (1105.3) and AI/AN persons (1024). [54]
Mortality and diabetes
Type 1 and type 2 diabetes are both independently associated with a significant increased odds of in-hospital death with COVID-19. In a nationwide analysis in England of 61,414,470 individuals in the registry alive as of February 19, 2020, 0.4% had a recorded diagnosis of type 1 diabetes and 4.7% of type 2 diabetes. A total of 23,804 COVID-19 deaths in England were reported as of May 11, 2020; one third were in people with diabetes, including 31.4% with type 2 diabetes and 1.5% with type 1 diabetes. Upon multivariate adjustment, the odds of in-hospital COVID-19 death were 3.5 for those with type 1 diabetes and 2.03 for those with type 2 diabetes, compared with deaths among individuals without known diabetes. Further adjustment for cardiovascular comorbidities found the odds ratios were still significantly elevated in both type 1 (2.86) and type 2 (1.81) diabetes. [94]
The CDC estimates diabetes is associated with a 20% increased odds of in-hospital mortality. [54]
Hospitalization and cardiometabolic conditions
O’Hearn et al estimate nearly 2 in 3 adults hospitalized for COVID-19 in the United States have associated cardiometabolic conditions including total obesity (BMI 30 kg/m2 or greater), diabetes mellitus, hypertension, and heart failure. [95]
Virology
The full genome of SARS-CoV-2 was first posted by Chinese health authorities soon after the initial detection, facilitating viral characterization and diagnosis. The CDC analyzed the genome from the first US patient who developed the infection on January 24, 2020, concluding that the sequence is nearly identical to the sequences reported by China. [1] SARS-CoV-2 is a group 2b beta-coronavirus that has at least 70% similarity in genetic sequence to SARS-CoV. [96] Like MERS-CoV and SARS-CoV, SARS-CoV-2 originated in bats. [1] Viral variants emerge when the virus develops one or more mutations that differentiate it from the predominant virus variants circulating in a population. The CDC surveillance of SARS-CoV-2 variants includes US COVID-19 cases caused by variants. The site also includes which mutations are associated with particular variants. The CDC has launched a genomic surveillance dashboard and a website tracking US COVID-19 case trends caused by variants. Researchers are studying how variants may or may not alter the extent of protection by available vaccines. For more information, see the Medscape topic COVID-19 Variants. Omicron The Omicron variant (B.1.1.529), initially identified in South Africa, was declared a variant of concern in the United States by the CDC November 30, 2021. This VOC contains several dozen mutations, including a large number in the spike gene, more than previous VOCs. These mutations include several associated with increased transmission. The Omicron variant quickly became dominant in the United States. As of January 8, 2022, it accounted for over 98% of circulating virus, compared with less than 8% on December 11, 2021. Antiviral agent effectiveness An in vitro study published in December 2021 indicate that remdesivir, nirmatrelvir, molnupiravir, EIDD-1931, and GS-441524 (oral prodrug of remdesivir) retain their activity against the VOCs Alpha, Beta, Gamma, Delta, and Omicron. [97] Vaccine effectiveness A preprinted, nonpeer reviewed article of routine surveillance data from South Africa suggests the Omicron variant may evade immunity from prior infection. Among 2,796,982 individuals with laboratory-confirmed SARS-CoV-2 who had a positive test result for SARS-CoV-2 at least 90 days before November 27, 2021, there were 35,670 suspected reinfections identified. [98] In another preprinted article, neutralization performed with sera from double or triple BNT162b2-vaccinated individuals (6, 0.5 or 3 months after last vaccination/booster) revealed an 11.4-, 37.0- and 24.5-fold reduction, respectively. Sera from double mRNA-1273-vaccinated and additionally BNT162b2-vaccinated individuals (sampled 6 or 0.5 months after last vaccination/booster) showed a 20- and 22.7-fold reduction in the neutralization capacity. [99] Delta The Delta variant (B.1.617.2) that was first identified in India became the dominant variant in the United States in mid-July 2021. This variant increases ACE binding and transmissibility. An approximate 6.8-fold decreased neutralization for mRNA vaccines and convalescent plasma was observed with the Delta variant. [100, 101] However, a study completed by Public Health England found the BNT162b2 vaccine was only slightly reduced from 93.7% with the B.1.1.7 variant to 88% for the Delta variant 2 weeks after the second dose. [102] As the Omicron variant transmission increased rapidly in December 2021, the Delta variant now accounts for less than 2% of cases in the United States. Alpha The CDC tracks variant proportions circulating in the United States and estimates the B.1.1.7 variant (Alpha) that was first detected in the United Kingdom accounted for over 44% of cases from January 2 to March 27, 2021. On April 7, 2021, the CDC announced B.1.1.7 was the dominant strain circulating in the United States. It was the dominant strain until mid-July 2021, when the Delta variant became the dominant strain. At the same time that the transmission of the wild type virus was dropping, the variant increased, suggesting that the same recommendations (eg, masks, social distancing) may not be enough. The UK variant is also infecting more children (aged 19 years and younger) than the wild type, indicating that it may be more transmissible in children. This has raised concerns because a relative sparing of children has been observed to date. This variant is hypothesized to have a stronger ACE binding than the original variant, which was felt to have trouble infecting younger individuals as they express ACE to a lesser degree. [103] Beta The E484K mutation was found initially in the South Africa VOC (B.1.351 [Beta]) and also with the Brazil variants in late 2020, and was observed in the UK variant in early February 2021. Position 484 and 501 mutations that are both present in the South African variant, and the combination is a concern that immune escape may occur. These mutations, among others, have combined to create the VOC B.1.351. [104] Gamma The Brazil VOC P.1 (Gamma) was responsible for an enormous second surge of infections. Sabino et al describe resurgence of COVID-19 in Manaus, Brazil in January 2021, despite a high seroprevalence. A study of blood donors indicated that 76% of the population had been infected with SARS-CoV-2 by October 2020. Hospitalizations for COVID-19 in Manaus numbered 3431 in January 1 to 19, 2021 compared with 552 for December 1 to 19, 2020. Hospitalizations had remained stable and low for 7 months prior to December 2020. Several postulated variables regarding this resurgence include waning titers to the original viral lineage and the high prevalence of the P.1 variant, which was first discovered in Manaus. [105] In addition, researchers are monitoring emergence of a second variant in Brazil, P.2, identified in Rio de Janeiro. As of September 21, 2021, the CDC lists P.2 as a variant being monitored. Epsilon VOCs B.1.427 (Epsilon) and B.1.429 (Epsilon) emerged in California. These variants accounted for 2.9% and 6.9% of variants circulating in the United States between January 2 to March 27, 2021. An approximate 20% increase in transmission has been observed with this variant.
Clinical Presentation
History
Presentations of COVID-19 range from asymptomatic/mild symptoms to severe illness and mortality. Common symptoms include fever, cough, and shortness of breath. [106] Other symptoms, such as malaise and respiratory distress, also have been described. [96]
Symptoms may develop 2 days to 2 weeks after exposure to the virus. [106] A pooled analysis of 181 confirmed cases of COVID-19 outside Wuhan, China, found the mean incubation period was 5.1 days, and that 97.5% of individuals who developed symptoms did so within 11.5 days of infection. [107]
Symptom rebound and viral rebound have been described in patients (with or without antiviral treatment). In untreated patients, those (n = 563) receiving placebo in the ACTIV-2/A5401 (Adaptive Platform Treatment Trial for Outpatients with COIVD-19) platform trial recorded 13 symptoms daily between days 1 and 28. Symptom rebound was identified in 26% of participants at a median of 11 days after initial symptom onset. Viral rebound was detected in 31% and high-level viral rebound in 13% of participants. [108]
The following symptoms may indicate COVID-19 [106] :
Fever or chills
Cough
Shortness of breath or difficulty breathing
Fatigue
Muscle or body aches
Headache
New loss of taste or smell
Sore throat
Congestion or runny nose
Nausea or vomiting
Diarrhea
Other reported symptoms include the following:
Sputum production
Malaise
Respiratory distress
Neurologic (eg, headache, altered mentality)
Wu and McGoogan reported that, among 72,314 COVID-19 cases reported to the CCDC, 81% were mild (absent or mild pneumonia), 14% were severe (hypoxia, dyspnea, >50% lung involvement within 24-48 hours), 5% were critical (shock, respiratory failure, multiorgan dysfunction), and 2.3% were fatal. [109] These general symptom distributions have been reconfirmed across multiple observations. [110, 111]
Clinicians evaluating patients with fever and acute respiratory illness should obtain information regarding travel history or exposure to an individual who recently returned from a country or US state experiencing active local transmission. [112]
Williamson and colleagues, in an analysis of 17 million patients, reaffirmed that severe COVID-19 and mortality was more common in males, older individuals, individuals in poverty, Black persons, and patients with medical conditions such as diabetes and severe asthma, among others. [113]
A multicenter observational cohort study conducted in Europe found frailty was a greater predictor of mortality than age or comorbidities. [114]
Type A blood has been suggested as a potential factor that predisposes to severe COVID-19, specifically in terms of increasing the risk for respiratory failure. Blood type O appears to confer a protective effect. [115, 116]
Patients with suspected COVID-19 should be reported immediately to infection-control personnel at their healthcare facility and the local or state health department. CDC guidance calls for the patient to be cared for with airborne and contact precautions (including eye shield) in place. [21] Patient candidates for such reporting include those with fever and symptoms of lower respiratory illness who have travelled from Wuhan City, China, within the preceding 14 days or who have been in contact with an individual under investigation for COVID-19 or a patient with laboratory-confirmed COVID-19 in the preceding 14 days. [112]
A complete or partial loss of the sense of smell (anosmia) has been reported as a potential history finding in patients eventually diagnosed with COVID-19. [19] A phone survey of outpatients with mildly symptomatic COVID-19 found that 64.4% (130 of 202) reported any altered sense of smell or taste. [117] In a European study of 72 patients with PCR results positive for COVID-19, 53 patients (74%) reported reduced olfaction, whereas 50 patients (69%) reported a reduced sense of taste. Forty-nine patients (68%) reported both symptoms. [118]
Physical Examination
Patients who are under investigation for COVID-19 should be evaluated in a private room with the door closed (an airborne infection isolation room is ideal) and asked to wear a surgical mask. All other standard contact and airborne precautions should be observed, and treating healthcare personnel should wear eye protection. [21]
The most common serious manifestation of COVID-19 upon initial presentation is pneumonia. Fever, cough, dyspnea, and abnormalities on chest imaging are common in these cases. [119, 120, 121, 122]
Huang and colleagues found that, among patients with pneumonia, 99% had fever, 70% reported fatigue, 59% had dry cough, 40% had anorexia, 35% experienced myalgias, 31% had dyspnea, and 27% had sputum production. [119]
Complications Complications of COVID-19 include pneumonia, acute respiratory distress syndrome, cardiac injury, arrhythmia, septic shock, liver dysfunction, acute kidney injury, and multi-organ failure, among others. Approximately 5% of patients with COVID-19, and 20% of those hospitalized, experience severe symptoms necessitating intensive care. The common complications among hospitalized patients include pneumonia (75%), ARDS (15%), AKI (9%), and acute liver injury (19%). Cardiac injury has been increasingly noted, including troponin elevation, acute heart failure, dysrhythmias, and myocarditis. Ten percent to 25 percent of hospitalized patients with COVID-19 experience prothrombotic coagulopathy resulting in venous and arterial thromboembolic events. Neurologic manifestations include impaired consciousness and stroke. ICU case fatality is reported up to 40%. [110] Long COVID As the COVID-19 pandemic has matured, more patients have reported long-term, post-infection sequelae. Most patients recover fully, but those who do not have reported adverse symptoms such as fatigue, dyspnea, cough, anxiety, depression, inability to focus (ie, “brain fog”), gastrointestinal problems, sleep difficulties, joint pain, and chest pain lasting weeks to months after the acute illness. Long-term studies are underway to understand the nature of these complaints. [123] Post-acute sequelae of SARS-CoV-2 (PASC) infection is the medical term for what is commonly called long COVID or "long haulers". The NIH includes discussion of persistent symptoms or organ dysfunction after acute COVID-19 within guidelines that discuss the clinical spectrum of the disease. [124] The UK National Institute for Health and Care Excellence (NICE) issued guidelines on care of long COVID that define the syndrome as: signs and symptoms that develop during or after an infection consistent with COVID-19, continue for more than 12 weeks, and are not explained by an alternative diagnosis. [125] Please see Long COVID-19. Future public health implications Public health implications for long COVID need to be examined, as reviewed by Datta et al. As with other infections (eg, Lyme disease, syphilis, Ebola), late inflammatory and virologic sequelae may emerge. Accumulation of evidence beyond the acute infection and postacute hyperinflammatory illness is important to evaluate to gain a better understanding of the full spectrum of the disease. [126] Thrombotic manifestations of severe COVID-19 are caused by the ability of SARS-CoV-2 to invade endothelial cells via angiotensin-converting enzyme-2 (ACE-2), which is expressed on the surface of endothelial cells. Subsequent endothelial inflammation, complement activation, thrombin generation, platelet and leukocyte recruitment, and the initiation of innate and adaptive immune responses culminate in immunothrombosis, and can ultimately cause microthrombotic complications (eg, DVT, PE, stroke). [127] Kotecha et al describe patterns of myocardial injury in hospitalized patients with severe COVID-19 who had elevated troponin levels. During convalescence, myocarditis-like injury was observed, with limited extent and minimal functional consequence. However, in a proportion of patients, there was evidence of possible ongoing localized inflammation. Roughly 25% of patients had ischemic heart disease, of which two thirds had no previous history. [128] Reinfection COVID-19 reinfection is defined as an infected person who has undergone full vaccination, whether they have had a booster or boosters. According to the CDC, reinfection is COVID-19 infection of an individual with 2 different viral strains that occurs at least 45 days apart. It also may occur when an individual has 2 positive CoV-2 RT-PCR tests with negative tests between the 2 positive tests. [129] It is essential to determine reinfection rates to establish the effectiveness of current vaccine prophylaxis. Reinfection in vaccinated and non-vaccinated persons probably is due to a variant. [129, 130] It is important to differentiate reinfection from reactivation or relapse of the virus, which occurs in a clinically recovered person within the first 4 weeks of infection, during which viral RNA testing has remained positive. During relapse, a tiny viral load of dormant virus reactivates, the reason of which often is unclear. The only way to prove this state is to show that genetic samples taken at the beginning and at the time of reactivation differ genetically; such testing is unusual at the beginning of a person’s illness.
Workup
Approach Considerations
Diagnostic testing for SARS-CoV-2 infection can be conducted by the CDC, state public health laboratories, hospitals using their own developed and validated tests, and some commercial reference laboratories. [131]
State health departments with a patient under investigation (PUI) should contact CDC’s Emergency Operations Center (EOC) at 770-488-7100 for assistance with collection, storage, and shipment of clinical specimens for diagnostic testing. Specimens from the upper respiratory tract, lower respiratory tract, and serum should be collected to optimize the likelihood of detection. [112]
The FDA now recommends that nasal swabs that access just the front of the nose be used in symptomatic patients, allowing for (1) a more comfortable and simplified collection method and (2) self-collection at collection sites. [132]
Various organizations, including the CDC, have published guidelines on COVID-19.
Laboratory Studies
Signs and symptoms of coronavirus disease 2019 (COVID-19) may overlap with those of other respiratory infections; therefore, it is important to perform laboratory testing to specifically identify symptomatic individuals infected with severe acute respiratory syndrome coronavirus 2 (SARS-CoV-2).
Three types of tests may be utilized to determine if an individual has been infected with SARS-CoV-2:
Viral nucleic acid (RNA) detection
Viral antigen detection
Detection of antibodies to the virus
Viral tests (nucleic acid or antigen detection tests) are used to assess acute infection, whereas antibody tests provide evidence of prior infection with SARS-CoV-2. Home sample collection kits for COVID-19 testing have been available by prescription; in December 2020, the LabCorp Pixel COVID-19 Test Home Collection Kit became the first to receive an FDA EUA for nonprescription use.
The FDA has advised against the use of antibody tests to ascertain immunity or protection from COVID-19, particularly in patients who have been vaccinated against the disease. According to the agency, differences between antibodies that arise from prior SARS-CoV-2 infection and those induced by vaccination leave the tests unable to determine whether an individual has achieved protection through a vaccine.
Laboratory findings in patients with COVID-19
Leukopenia, leukocytosis, and lymphopenia were common among early cases. [96, 119]
Lactate dehydrogenase and ferritin levels are commonly elevated. [119]
Wu and colleagues [133] reported that, among 200 patients with COVID-19 who were hospitalized, older age, neutrophilia, and elevated lactate dehydrogenase and D-dimer levels increased the risks for ARDS and death.
CT Scanning
Chest computed tomography (CT) scanning in patients with COVID-19–associated pneumonia usually shows ground-glass opacification, possibly with consolidation. Some studies have reported that abnormalities on chest CT scans are usually bilateral, involve the lower lobes, and have a peripheral distribution. Pleural effusion, pleural thickening, and lymphadenopathy have also been reported, although with less frequency. [119, 134, 135]
Bai and colleagues reported the following common chest CT scanning features among 201 patients with CT abnormalities and positive RT-PCR results for COVID-19 [136] :
Peripheral distribution (80%)
Ground-glass opacity (91%)
Fine reticular opacity (56%)
Vascular thickening (59%)
Less-common features on chest CT scanning included the following [136] :
Central and peripheral distribution (14%)
Pleural effusion (4.1%)
Lymphadenopathy (2.7%)
The American College of Radiology (ACR) recommends against using CT scanning for screening or diagnosis but instead reserving it for management in hospitalized patients. [137]
At least two studies have reported on manifestations of infection in apparently asymptomatic individuals. Hu and colleagues reported on 24 asymptomatic infected persons in whom chest CT scanning revealed ground-glass opacities/patchy shadowing in 50% of cases. [138] Wang and colleagues reported on 55 patients with asymptomatic infection, two thirds of whom had evidence of pneumonia as revealed by CT scanning. [139]
Progression of CT abnormalities
Li and colleagues recommend high-resolution CT scanning and reported the following CT changes over time in patients with COVID-19 among three Chinese hospitals:
Early phase: Multiple small patchy shadows and interstitial changes begin to emerge in a distribution beginning near the pleura or bronchi rather than the pulmonary parenchyma.
Progressive phase: The lesions enlarge and increase, evolving to multiple ground-glass opacities and infiltrating consolidation in both lungs.
Severe phase: Massive pulmonary consolidations occur, while pleural effusion is rare.
Dissipative phase: Ground-glass opacities and pulmonary consolidations are absorbed completely. The lesions begin evolving into fibrosis. [140]
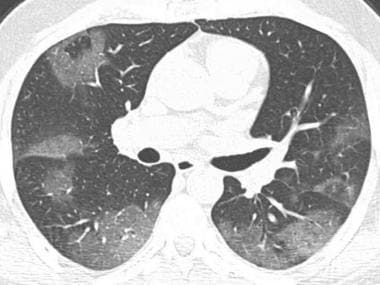
Axial chest CT demonstrates patchy ground-glass opacities with peripheral distribution.
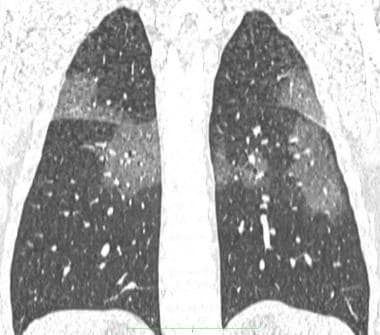
Coronal reconstruction chest CT of the same patient above, showing patchy ground-glass opacities.
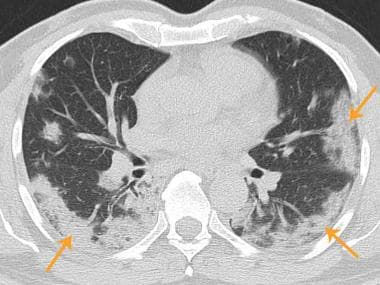
Axial chest CT shows bilateral patchy consolidations (arrows), some with peripheral ground-glass opacity. Findings are in peripheral and subpleural distribution.
Chest Radiography
In a retrospective study of patients in Hong Kong with COVID-19, common abnormalities on chest radiography, when present, included consolidation (30 of 64 patients; 47%) and ground-glass opacities (33%). Consolidation was commonly bilateral and of lower zone distribution. Pleural effusion was an uncommon finding. Severity on chest radiography peaked 10 to 12 days after symptom onset. [141]
Chest radiography may reveal pulmonary infiltrates. [142]
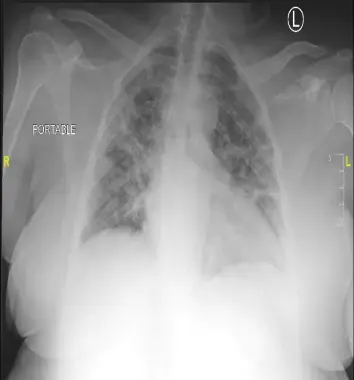
The heart is normal in size. There are diffuse, patchy opacities throughout both lungs, which may represent multifocal viral/bacterial pneumonia versus pulmonary edema. These opacities are particularly confluent along the periphery of the right lung. There is left midlung platelike atelectasis. Obscuration of the left costophrenic angle may represent consolidation versus a pleural effusion with atelectasis. There is no pneumothorax.
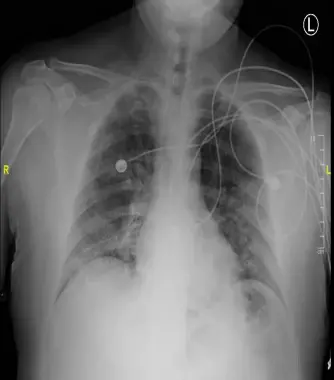
The heart is normal in size. There are bilateral hazy opacities, with lower lobe predominance. These findings are consistent with multifocal/viral pneumonia. No pleural effusion or pneumothorax are seen.

The heart is normal in size. Patchy opacities are seen throughout the lung fields. Patchy areas of consolidation at the right lung base partially silhouettes the right diaphragm. There is no effusion or pneumothorax. Degenerative changes of the thoracic spine are noted.

The same patient as above 10 days later.

The trachea is in midline. The cardiomediastinal silhouette is normal in size. There are diffuse hazy reticulonodular opacities in both lungs. Differential diagnoses include viral pneumonia, multifocal bacterial pneumonia or ARDS. There is no pleural effusion or pneumothorax.
Treatment & Management
Approach Considerations
Utilization of programs established by the FDA to allow clinicians to gain access to investigational therapies during the pandemic has been essential. The expanded access (EA) and emergency use authorization (EUA) programs allowed for rapid deployment of potential therapies for investigation and investigational therapies with emerging evidence. A review by Rizk et al describes the role for each of these measures, and their importance to providing medical countermeasures in the event of infectious disease and other threats. [22]
Remdesivir, an antiviral agent, was the first drug to gain full FDA approval for treatment of hospitalized adults and adolescents with COVID-19 disease in October 2020. [23] Since then, it has gained approval for adults and pediatric patients (aged 28 days and older who weigh at least 3 kg) with positive results of direct SARS-CoV-2 viral testing, who are hospitalized, or not hospitalized and have mild-to-moderate COVID-19, and are at high risk for progression to severe COVID-19, including hospitalization or death. [24]
Treatment does not preclude isolation and masking for those who test positive for SARS-CoV-2.
The first vaccine to gain full FDA approval was mRNA-COVID-19 vaccine (Comirnaty; Pfizer) in August 2021. A second mRNA vaccine (Spikevax; Moderna) was approved by the FDA in January 2022. Additionally, each of these vaccines have EUAs for children as young as 6 months.
Baricitinib (Olumiant), a Janus kinase inhibitor, gained FDA approval for hospitalized adults with COVID-19 disease who require supplemental oxygen, noninvasive or invasive mechanical ventilation, or ECMO. An EUA for children has been issued for baricitinib.
Similar to baricitinib, tocilizumab (Actemra), an interleukin 6 inhibitor, was approved by the FDA for hospitalized adults. An EUA remains in place for children aged 2 years and older.
EUAs have also been issued for vaccines and convalescent plasma in the United States. A full list of EUAs and access to the Fact Sheets for Healthcare Providers are available from the FDA.
Use of corticosteroids improves survival in hospitalized patients with severe COVID-19 disease requiring supplemental oxygen, with the greatest benefit shown in those requiring mechanical ventilation. [25]
All infected patients should receive supportive care to help alleviate symptoms. Vital organ function should be supported in severe cases. [13]
Early in the outbreak, concerns emerged about nonsteroidal anti-inflammatory drugs (NSAIDs) potentially increasing the risk for adverse effects in individuals with COVID-19. However, in late April 2020, the WHO took the position that NSAIDS do not increase the risk for adverse events or affect acute healthcare utilization, long-term survival, or quality of life. [143]
Numerous collaborative efforts to discover and evaluate effectiveness of antivirals, immunotherapies, monoclonal antibodies, and vaccines have rapidly emerged. Guidelines and reviews of pharmacotherapy for COVID-19 have been published. [26, 27, 28, 29] The Milken Institute maintains a detailed COVID-19 Treatment and Vaccine Tracker of research and development progress.
Searching for effective therapies for COVID-19 infection is a complex process. Gordon and colleagues identified 332 high-confidence SARS-CoV-2 human protein-protein interactions. Among these, they identified 66 human proteins or host factors targeted by 69 existing FDA-approved drugs, drugs in clinical trials, and/or preclinical compounds. As of March 22, 2020, these researchers are in the process of evaluating the potential efficacy of these drugs in live SARS-CoV-2 infection assays. [144]
The NIH Accelerating Covid-19 Therapeutics Interventions and Vaccines (ACTIV) trials public-private partnership to develop a coordinated research strategy has several ongoing protocols that are adaptive to the progression of standard care.
How these potential COVID-19 treatments will translate to human use and efficacy is not easily or quickly understood. The question of whether some existing drugs that have shown in vitro antiviral activity might achieve adequate plasma pharmacokinetics with current approved doses was examined by Arshad and colleagues. The researchers identified in vitro anti–SARS-CoV-2 activity data from all available publications up to April 13, 2020, and recalculated an EC90 value for each drug. EC90 values were then expressed as a ratio to the achievable maximum plasma concentrations (Cmax) reported for each drug after administration of the approved dose to humans (Cmax/EC90 ratio). The researchers also calculated the unbound drug to tissue partition coefficient to predict lung concentrations that would exceed their reported EC50 levels. [145]
The WHO developed a blueprint of potential therapeutic candidates in January 2020. The WHO embarked on an ambitious global "megatrial" called SOLIDARITY in which confirmed cases of COVD-19 are randomly assigned to standard care or 1 of 4 active treatment arms (remdesivir, chloroquine or hydroxychloroquine, lopinavir/ritonavir, or lopinavir/ritonavir plus interferon beta-1a). In early July 2020, the treatment arms in hospitalized patients that included hydroxychloroquine, chloroquine, or lopinavir/ritonavir were discontinued owing to the drugs showing little or no reduction in mortality compared with standard of care. [146] Interim results released mid-October 2020 found the 4 aforementioned repurposed antiviral agents appeared to have little or no effect on hospitalized patients with COVID-19, as indicated by overall mortality, initiation of ventilation, and duration of hospital stay. The 28-day mortality was 12% (39% if already ventilated at randomization, 10% otherwise). [147]
The next phase of the trial, Solidarity PLUS, continued in August 2021. WHO announced over 600 hospitals in 52 countries will participate in testing three drugs (ie, artesunate, imatinib, infliximab). Patients will be randomized to standard of care (SOC) or SOC plus one of the study drugs. The drugs for the trial were donated by the manufacturers; however, approximate costs are $400/day for imatinib, $3,500 for a dose of infliximab, and $50,000 for a course of artesunate.
The urgent need for treatments during a pandemic can confound the interpretation of resulting outcomes of a therapy if data are not carefully collected and controlled. Andre Kalil, MD, MPH, writes of the detriment of drugs used as a single-group intervention without a concurrent control group that ultimately lead to no definitive conclusion of efficacy or safety. [148]
Rome and Avorn write about unintended consequences of allowing widening access to experimental therapies. First, efficacy is unknown and may be negligible, but, without appropriate studies, physicians will not have evidence on which to base judgement. Existing drugs with well-documented adverse effects (eg, hydroxychloroquine) subject patients to these risks without proof of clinical benefit. Expanded access of unproven drugs may delay implementation of randomized controlled trials. In addition, demand for unproven therapies can cause shortages of medications that are approved and indicated for other diseases, thereby leaving patients who rely on these drugs for chronic conditions without effective therapies. [149]
Drug shortages during the pandemic go beyond off-label prescribing of potential treatments for COVID-19. Drugs that are necessary for ventilated and critically ill patients and widespread use of inhalers used for COPD or asthma are in demand. [150, 151]
It is difficult to carefully evaluate the onslaught of information that has emerged regarding potential COVID-19 therapies within a few months’ time in early 2020. A brief but detailed approach regarding how to evaluate resulting evidence of a study has been presented by F. Perry Wilson, MD, MSCE. By using the example of a case series of patients given hydroxychloroquine plus azithromycin, Wilson provides clinicians with a quick review of critical analyses. [152]
Ventilator application techniques
Ventilator management and monitoring
Respiratory conditions assessment and management
Prevention
The first vaccine to gain full FDA approval was mRNA-COVID-19 vaccine (Comirnaty; Pfizer) in August 2021. A second mRNA vaccine (Spikevax; Moderna) was approved by the FDA in January 2022. Additionally, each of these vaccines have EUAs for children as young as 6 months. EUAs have been issued for other vaccines.
Avoidance is the principal method of deterrence.
General measures for prevention of viral respiratory infections include the following [13] :
Handwashing with soap and water for at least 20 seconds. An alcohol-based hand sanitizer may be used if soap and water are unavailable.
Individuals should avoid touching their eyes, nose, and mouth with unwashed hands.
Individuals should avoid close contact with sick people.
Sick people should stay at home (eg, from work, school).
Coughs and sneezes should be covered with a tissue, followed by disposal of the tissue in the trash.
Frequently touched objects and surfaces should be cleaned and disinfected regularly.
Preventing/minimizing community spread of COVID-19
The CDC has recommended the below measures to mitigate community spread. [9, 153, 154]
All individuals in areas with prevalent COVID-19 should be vigilant for potential symptoms of infection and should stay home as much as possible, practicing social distancing (maintaining a distance of 6 feet from other persons) when leaving home is necessary.
Persons with an increased risk for infection—(1) individuals who have had close contact with a person with known or suspected COVID-19 or (2) international travelers (including travel on a cruise ship)—should observe increased precautions. These include (1) self-quarantine for at least 2 weeks (14 days) from the time of the last exposure and distancing (6 feet) from other persons at all times and (2) self-monitoring for cough, fever, or dyspnea with temperature checks twice a day.
On April 3, 2020, the CDC issued a recommendation that the general public, even those without symptoms, should begin wearing face coverings in public settings where social-distancing measures are difficult to maintain in order to abate the spread of COVID-19. [9]
Facemasks
In a 2020 study on the efficacy of facemasks in preventing acute respiratory infection, surgical masks worn by patients with such infections (rhinovirus, influenza, seasonal coronavirus [although not SARS-CoV-2 specifically]) were found to reduce the detection of viral RNA in exhaled breaths and coughs. Specifically, surgical facemasks were found to significantly decreased detection of coronavirus RNA in aerosols and influenza virus RNA in respiratory droplets. The detection of coronavirus RNA in respiratory droplets also trended downward. Based on this study, the authors concluded that surgical facemasks could prevent the transmission of human coronaviruses and influenza when worn by symptomatic persons and that this may have implications in controlling the spread of COVID-19. [155]
In a 2016 systematic review and meta-analysis, Smith and colleagues found that N95 respirators did not confer a significant advantage over surgical masks in protecting healthcare workers from transmissible acute respiratory infections. [156]
Investigational agents for postexposure prophylaxis
PUL-042
PUL-042 (Pulmotech, MD Anderson Cancer Center, and Texas A&M) is a solution for nebulization with potential immunostimulating activity. It consists of two toll-like receptor (TLR) ligands: Pam2CSK4 acetate (Pam2), a TLR2/6 agonist, and the TLR9 agonist oligodeoxynucleotide M362.
PUL-042 binds to and activates TLRs on lung epithelial cells. This induces the epithelial cells to produce peptides and reactive oxygen species (ROS) against pathogens in the lungs, including bacteria, fungi, and viruses. M362, through binding of the CpG motifs to TLR9 and subsequent TLR9-mediated signaling, initiates the innate immune system and activates macrophages, natural killer (NK) cells, B cells, and plasmacytoid dendritic cells; stimulates interferon-alpha production; and induces a T-helper 1 cells–mediated immune response. Pam2CSK4, through TLR2/6, activates the production of T-helper 2 cells, leading to the production of specific cytokines. [157]
In May 2020, the FDA approved initiation of two COVID-19 phase 2 clinical trials of PUL-042 at up to 20 US sites. The trials are for the prevention of infection with SARS-CoV-2 and the prevention of disease progression in patients with early COVID-19. In the first study, up to 4 doses of PUL-042 or placebo will be administered to 200 participants via inhalation over a 10-day period to evaluate the prevention of infection and reduction in severity of COVID-19. In the second study, 100 patients with early symptoms of COVID-19 will receive PUL-042 up to 3 times over 6 days. Each trial will monitor participants for 28 days to assess effectiveness and tolerability. [158, 159]
Antiviral Agents Remdesivir Remdesivir (Veklury) was the first drug approved by the FDA for treating the SARS-CoV-2 virus. It is indicated for treatment of COVID-19 disease in hospitalized adults and children aged 12 years and older who weigh at least 40 kg. The broad-spectrum antiviral is a nucleotide analog prodrug. [23] Full approval was preceded by the US FDA issuing an EUA (emergency use authorization) on May 1, 2020. [160] Upon approval of remdesivir in adults and adolescents, the EUA was updated to maintain the ability for prescribers to treat pediatric patients weighing 3.5 kg to less than 40 kg or children younger than 12 years who weigh at least 3.5 kg. [24] The remdesivir EUA was expanded to include moderate disease August 28, 2020. This expands the previous authorization to treat all hospitalized patients with COVID-19 regardless of oxygen status. [161] A new drug application (NDA) for remdesivir was submitted to the FDA in August 2020. A phase 1b trial of an inhaled nebulized version was initiated in late June 2020 to determine if remdesivir can be used on an outpatient basis and at earlier stages of disease. [162] As of October 1, 2020, remdesivir is available from the distributor (ie, AmerisourceBergen). Wholesale acquisition cost is approximately $520/100-mg vial, totaling $3,120 for a 5-day treatment course. Inpatient remdesivir Several phase 3 clinical trials have tested remdesivir for treatment of COVID-19. Positive results were seen with remdesivir after use by the University of Washington in the first case of COVID-19 documented on US soil in January 2020. [163] An adaptive randomized trial of remdesivir coordinated by the National Institute of Health (NCT04280705) was started first against placebo, but additional therapies were added to the protocol as evidence emerged and treatment evolved. The first experience with this study involved passengers of the Diamond Princess cruise ship in quarantine at the University of Nebraska Medical Center in February 2020 after returning to the United States from Japan following an on-board outbreak of COVID-19. [164] Trials of remdesivir for moderate and severe COVID-19 compared with standard of care and varying treatment durations are ongoing. The initial EUA for remdesivir was based on preliminary data analysis of the Adaptive COVID-19 Treatment Trial (ACTT), and was announced April 29, 2020. The final analysis included 1,062 hospitalized patients with advanced COVID-19 and lung involvement, showing that patients treated with 10-days of remdesivir had a 31% faster time to recovery than those who received placebo (remdesivir, 10 days; placebo, 15 days; P < 0.001). Patients with severe disease (n = 957) had a median time to recovery of 11 days compared with 18 days for placebo. A statistically significant difference was not reached for mortality by day 15 (remdesivir 6.7% vs placebo 11.9%) or by day 29 (remdesivir 11.4% vs placebo 15.2%). [165] The final ACTT-1 results for shortening the time to recovery differed from interim results from the WHO SOLIDARITY trial for remdesivir. These discordant conclusions are complicated and confusing as the SOLIDARITY trial included patients from ACTT-1. [147] An editorial by Harrington and colleagues [166] notes the complexity of the SOLIDARITY trial and the variation within and between countries in the standard of care and in the burden of disease in patients who arrive at hospitals. The authors also mention that trials solely focused on remdesivir were able to observe nuanced outcomes (ie, ability to change the course of hospitalization), whereas the larger, simple randomized SOLIDARITY trial focused on more easily defined outcomes. Similar to the SOLIDARITY trial, the DisCoVeRy open-labeled, multicenter trial did not show clinical benefit from use of remdesivir. The trial was conducted in 48 sited throughout Europe from March 22, 2020 to January 21, 2022. However, among the participants included in the SOLIDARITY trial, 219 (8%) of 2750 participants who were randomly assigned to receive remdesivir and 221 (5.4%) of 4088 randomly assigned to standard of care were shared by the DisCoVeRy trial. These shared patients between the 2 trials accounted for approximately 50% of DisCoVeRy participants (remdesivir plus SOC [n = 429]; SOC alone [n = 428]). Standard of care did not include dexamethasone until October 2021 in this trial. [167] The open-label phase 3 SIMPLE trial (n = 397) in hospitalized patients with severe COVID-19 disease not requiring mechanical ventilation showed similar improvement in clinical status with the 5-day remdesivir regimen compared with the 10-day regimen on Day 14 (odds ratio, 0.75). After adjustment for imbalances in baseline clinical status, patients receiving a 10-day course of remdesivir had a distribution in clinical status at Day 14 that was similar to that of patients receiving a 5-day course (P = 0.14). The findings could significantly expand the number of patients who could be treated with the current supply of remdesivir. The trial is continuing with an enrollment goal of 6,000 patients. [168] Similarly, the phase 3 SIMPLE II trial in patients with moderate COVID-19 disease (n = 596) showed that 5 days of remdesivir treatment had a statistically significant higher odds of a better clinical status distribution on Day 11 compared with those receiving standard care (odds ratio, 1.65; P = 0.02). Improvement on Day 11 did not differ between the 10-day remdesivir group and standard of care group (P = 0.18). [169] The phase 3 PINETREE trial evaluated remdesivir as a 3-day outpatient regimen in high-risk patients with COVID-19. An analysis of 562 patients showed an 87% reduction in risk for COVID-19 related hospitalization or all-cause death by Day 28 for remdesivir (0.7% [2/279]) compared with placebo (5.3% [15/283]) P = 0.008. Remdesivir was also associated with an 81% reduction in the risk for medical visits owing to COVID-19 or all-cause death (1.6% vs 8.3% with placebo; P = 0.002). [170] Real-world analysis Three retrospective real-world studies presented at the 30th Conference on Retroviruses and Opportunistic Infections (CROI) 2023 showed when remdesivir was initiated within the first 2 days of hospital admission, patients had significantly lower risk for mortality and hospital readmission compared with matched controls. The studies included more than 500,000. [171] Two studies analyzed clinical practice information from the US Premier Healthcare databases of more than 500,000 adult patients hospitalized with COVID-19. The overall analysis examined all-cause inpatient mortality rates at 14- and 28- days and demonstrated that initiation of remdesivir within the first 2 days of hospital admission was associated with a statistically significant lower risk for mortality in all oxygen levels compared to matched controls that did not receive remdesivir during their hospitalization. For patients with no documented use of supplemental oxygen at baseline, treatment with remdesivir was associated with a 19% (P< 0.001) lower risk of mortality at Day 28. Patients on low-flow or high-flow oxygen also had a 21% (P< 0.001) and 12% (P< 0.001) lower risk of mortality at Day 28, respectively. Patients on invasive mechanical ventilation/ECMO at baseline had a 26% (P< 0.001) reduced risk for mortality at Day 28. The second analysis demonstrated that a reduction in mortality was also associated in vulnerable patient populations (eg, patients with immunocompromised conditions, who can experience repeat infections and breakthrough infections). Results demonstrated that at Day 28 mortality results showed that timely initiation of remdesivir treatment within 2 days of hospital admission was associated with an overall 25% significantly lower risk compared to non-remdesivir across all variant time periods, ie, pre-Delta (35%), Delta (21%), Omicron (16%). A meta-analysis showed remdesivir reduced mortality in patients hospitalised with COVID-19 who required no or conventional oxygen support, but was underpowered to evaluate patients who were ventilated when receiving remdesivir. [172] Remdesivir use in children Remdesivir emergency use authorization includes pediatric dosing that was derived from pharmacokinetic data in healthy adults. Remdesivir has been available through compassionate use to children with severe COVID-19 since February 2020. A phase 2/3 trial (CARAVAN) of remdesivir was initiated in June 2020 to assess safety, tolerability, pharmacokinetics, and efficacy in children with moderate-to-severe COVID-19. CARAVAN is an open-label, single-arm study of remdesivir in children from birth to age 18 years. [173] Data were presented on compassionate use of remdesivir in children at the virtual COVID-19 Conference held July 10-11, 2020. Most of the 77 children with severe COVID-19 improved with remdesivir. Clinical recovery was observed in 80% of children on ventilators or ECMO and in 87% of those not on invasive oxygen support. [174] Remdesivir use in pregnant females Outcomes in the first 86 pregnant women who were treated with remdesivir (March 21 to June 16, 2020) found high recovery rates. Recovery rates were high among women who received remdesivir (67 while pregnant and 19 on postpartum days 0-3). No new safety signals were observed. At baseline, 40% of pregnant individuals (median gestational age, 28 weeks) required invasive ventilation compared with 95% of postpartum patients (median gestational age at delivery 30 weeks). Among pregnant patients, 93% of those on mechanical ventilation were extubated, 93% recovered, and 90% were discharged. Among postpartum individuals, 89% were extubated, 89% recovered, and 84% were discharged. There was 1 maternal death attributed to underlying disease and no neonatal deaths. [175] Data continue to emerge. A case series of 5 patients describes successful treatment and monitoring throughout treatment with remdesivir in pregnant women with COVID-19. [176] Outpatient remdesivir Remdesivir gained approval from the FDA for outpatient use in nonhospitalized adults and pediatric patients aged 12 years and older who weigh at least 40 kg with mild-to-moderate COVID-19 who are at high risk for progression to severe disease, including hospitalization or death. Additionally, the EUA for younger children and those weighing less than 40 kg was amended to include outpatient use for mild-to-moderate disease in high-risk individuals. Results from the randomized, double-blind, placebo-controlled PINETREE trial supported the expanded indication and EUA. Among 562 outpatients with COVID-19 at high risk for disease progression demonstrated an 87% lower risk of hospitalization or death compared with than placebo (p = 0.008). Overall, 2 of 279 patients who received remdesivir (0.7%) required COVID-19 related hospitalization compared with 15 of 283 patients who received a placebo (5.3%). The study included patients who tested positive for SARS-CoV-2 with symptom onset within the previous 7 days and at least 1 risk factor for disease progression. Patients received either 3 consecutive days of IV remdesivir (200 mg IV on Day 1, then 100 mg on Days 2 and 3) or placebo. [170] The outpatient remdesivir dose for children weighing less than 40 kg is 5 mg/kg IV on Day 1, then 2.5 mg/kg
Symptom/viral rebound
Concerns regarding antiviral agents, particularly nirmatrelvir/ritonavir (Paxlovid), causing rebound of symptoms were vocalized in the press and social media. The course of viral infections with fluctuating viral loads and symptoms is not unique to SARS-CoV 2. However, studies have shown no difference in risk of viral rebound among nirmatrelvir/ritonavir compared with control groups that included usual care, placebo, and/or another drug (eg, other antiviral agent, monoclonal antibodies). [177, 178]
Symptom rebound and viral rebound has been described in patients with COVID-19 (with or without antiviral treatment). In untreated patients (n = 563) receiving placebo in the ACTIV-2/A5401 (Adaptive Platform Treatment Trial for Outpatients with COIVD-19) platform trial recorded 13 symptoms daily between Days 1 and 28. Symptom rebound was identified in 26% of participants at a median of 11 days after initial symptom onset. Viral rebound was detected in 31% and high-level viral rebound in 13% of participants. [108]
Nirmatrelvir/ritonavir
Nirmatrelvir/ritonavir (Paxlovid) was granted an EUA December 22, 2021 for treatment of mild-to-moderate COVID-19 in adults and pediatric patients aged 12 years and older who weigh at least 40 kg and are at high risk for progression to severe COVID-19, including hospitalization or death. Nirmatrelvir inhibits SARS-CoV2-3CL protease, and thereby inhibits viral replication at the proteolysis stage (ie, before viral RNA replication). Nirmatrelvir is combined with low-dose ritonavir to slow its metabolism and provide higher systemic exposure.
Results from the phase 2/3 trial Evaluation of Protease Inhibition for COVID-19 in nonhospitalized high-risk adults (EPIC-HR) (n = 2,246) showed a relative risk reduction for hospitalization or death by 89.1% with nirmatrelvir plus ritonavir when initiated within 3 days of symptom onset and 88% when initiated within 5 days of symptom onset compared with placebo. Hospitalization through Day 28 among patients who received nirmatrelvir/ritonavir within 3 days was 0.7% (5/697 hospitalized with no deaths), compared with 6.5% of patients who received placebo and were hospitalized or died (44/682 hospitalized with 9 subsequent deaths) (P< 0.0001). Similarly, patients who received nirmatrelvir/ritonavir within 5 days had a reduced risk for hospitalization or death for any cause by 88% compared with placebo (P < 0.0001). [179]
The EPIC-SR (standard risk adults) included unvaccinated adults who were at standard risk as well as vaccinated adults who had 1 or more risk factors for progressing to severe illness. Interim analysis showed 0.6% of patients were hospitalized compared with 2.4% in the placebo group, a 70% reduction in hospitalization and no deaths in the treated population. [180]
Another clinical trial, EPIC-PEP (Post-Exposure Prophylaxis), administers nirmatrelvir/ ritonavir as postexposure prophylaxis to adult household contacts living with an individual with a confirmed symptomatic SARS-COV-2 infection. [181]
In a retrospective study conducted by the Missouri Veterans Affairs, outpatients treated with nirmatrelvir/ritonavir within 5 days of testing positive for COVID-19 (n = 9,217) reduced the risk of long COVID compared with untreated outpatients (n = 47,123). [182]
An open-label, multicenter, randomized trial determined nirmatrelvir/ritonavir did not reduce the risk of all-cause mortality on day 28 in hospitalized adults. Criteria included hospitalized adults with severe comorbidities, confirmed SARS-CoV-2 infection by positive of real-time PCR within the previous 48 hours, and duration from symptoms onset to hospital admission less than 5 days. [183]
Molnupiravir
An EUA for molnupiravir was granted on December 23, 2021 for treatment of mild-to-moderate COVID-19 in adults aged 18 years and older and are at high risk for progression to severe COVID-19, including hospitalization or death.
Molnupiravir (MK-4482 [previously EIDD-2801]; Merck) is an oral antiviral agent that is a prodrug of the nucleoside derivative N4-hydroxycytidine. It elicits antiviral effects by introducing copying errors during viral RNA replication of the SARS-CoV-2 virus.
The phase 3 outpatient MOVe-OUT study (n = 1433) found molnupiravir reduced risk for hospitalization or death from 9.7% (68 of 699) in the placebo group to 6.8% (48 of 709) in the molnupiravir group for an absolute risk reduction of 3% (P = 0.02) and a relative risk reduction of 30%. Nine deaths were reported in the placebo group and one in the molnupiravir group. These data are consistent with the interim analysis. [184]
A real world analysis among United States veterans between January 5th and September 30th 2022 utilized medical records to measure hospital admission or death at 30 days in patients who received molnupiravir (N = 85,998) or no treatment (N = 78,180). Molnupiravir was associated with a reduction in hospital admissions or death at 30 days (relative risk 0.72) compared with no treatment; the event rates for hospital admission or death at 30 days were 2.7% for molnupiravir and 3.8% for no treatment; the absolute risk reduction was 1.1%. [185]
An earlier population-based real-world data from the largest healthcare provider in Israel was analyzed to evaluate molnupiravir efficacy. The study identify 2,661 adults with a first-ever positive test for severe acute respiratory syndrome coronavirus 2 (SARS-CoV-2) performed in the community during January–February 2022, who were at high risk for severe COVID-19. Study outcomes were defined as the composite of severe COVID-19 or COVID-19-specific mortality, specifically – O2 sat < 94% on room air, PaO2 < 300 mmHg, or RR >30 bm. Molnupiravir was associated with a nonsignificant reduced risk of the composite outcome. However, subgroup analyses showed that molnupiravir was associated with a significant decrease in risk of the composite outcome in older patients, in females, and in patients with inadequate COVID-19 vaccination. The results were similar when each component of the composite outcome was examined separately. [186]
Molnupiravir was evaluated in a phase 3 trial for postexposure prophylaxis for individuals residing in the same household with someone who tests positive for SARS-CoV-2 in the phase 3 MOVE-AHEAD trial. Molnupiravir did not demonstrate a statistically significant reduction in the risk of COVID-19 following household exposure. [187]
Investigational Antivirals
Obeldesivir
Obeldesivir (GS-5245; Gilead) is an isobutyl ester prodrug of GS-441524. Obeldesivir is hydrolyzed to its parent nucleoside, GS-441524, which is in turn converted to remdesivir-triphosphate (GS-443902). The isobutyl ester provides improved bioavailability for oral administration. It targets virus replication by inhibiting RNA polymerase. [188]
Ensitrelvir
Ensitrelvir fumaric acid (Shionogi Pharmaceuticals) is an oral 3CL protease inhibitor. SARS-CoV-2 has an enzyme called 3CL protease, which is essential for the replication of the virus. Ensitrelvir suppresses the replication of SARS-CoV-2 by selectively inhibiting 3CL protease. The phase 3 SCORPIO-SR study evaluated efficacy and safety in patients with mild-to-moderate COVID-19 in Japan, South Korea, and Vietnam. Patients received either ensitrelvir 125 mg orally (with an initial loading 375-mg dose) (n = 603) or placebo (n = 600) once daily for 5 days. Treatment was initiated within 5 days of onset of symptoms. Results showed a statistically significant reduction in time to resolution of symptoms (167.9 h vs 192.2 h; P = 0.0407). Additionally, a significant reduction in time to achieve negative infectious viral titer was observed with ensitrelvir compared with placebo (36.2 h vs 65.3 h; P < 0.0001). [189]
Bucillamine
Bucillamine (Revive Therapeutics) is an oral drug with anti-inflammatory and antiviral properties. It is derived from tiopronin and has been available in Japan and South Korea for over 30 years. N-acetyl-cysteine (NAC) has been shown to significantly attenuate clinical symptoms in respiratory viral infections in animals and humans, primarily via donation of thiols to increase antioxidant activity of cellular glutathione. Bucillamine has 2 thiol groups and its ability as a thiol donor is estimated to be 16 times that of NAC. A phase 3 trial for treatment of outpatients with mild-to-moderate COVID-19 at 40 sites in the United States is ongoing with an enrollment goal of 1000 participants. [190]
The SARS-CoV-2 Omicron variants have shown to be vulnerable to reduction. Bucillamine’s antioxidant effect demonstrated to have the most potent effect on inhibiting Omicron variants BA.1, BA.2, and BA.4/5 spike proteins. [191]
VV116
VV116 is an oral nucleoside antiviral drug shown to be noninferior to nirmatrelvir/ritonavir in a phase 3 study. VV116 is a deuterated remdesivir hydrobromide with oral bioavailability. Unlike nirmatrelvir/ritonavir, VV116 does not inhibit or induce major CYP enzymes or transporters, so drug interactions are less likely. [192]
Opaganib
Opaganib (Yeliva; RedHill Biopharma Ltd) is an orally administered sphingosine kinase-2 (SK2) inhibitor that may inhibit viral replication and reduce levels of IL-6 and TNF-alpha. Nonclinical data indicate both antiviral and anti-inflammatory effects. [193] A recently completed multinational phase 2/3 clinical trial of opaganib in patients hospitalized with COVID-19 demonstrated that opaganib resulted in a 62% decrease in mortality in a large subpopulation of patients with moderately severe Covid-19. [194]
Sabizabulin
Sabizabulin (VERU-111; Veru, Inc) is an oral microtubule depolymerization agent that has broad antiviral activity and has strong anti-inflammatory effects. [195] A phase 3 trial evaluated sabizabulin in hospitalized patients with moderate-to-severe COVID-19 who were at high risk for ARDS and death. Patients received sabizabulin or placebo in addition to SoC that included remdesivir, dexamethasone, ANI-IL6 receptor antibodies, and JAK inhibitors. Sabizabulin treatment resulted in a 24.9% absolute reduction and a 55.2% relative reduction in deaths compared with placebo(P = 0.0042). Sabizabulin treatment resulted in a 43% relative reduction in ICU days (p = 0.0013), a 49% relative reduction in days on mechanical ventilation (P = 0.0013), and a 26% relative reduction in days in the hospital (P = 0.0277) compared with placebo. [196]
Bemnifosbuvir
Bemnifosbuvir (AT-527; Atea Pharmaceuticals) is an oral purine nucleotide prodrug designed to inhibit RNA polymerase enzyme. It has demonstrated in vitro and in vivo antiviral activity against several enveloped single-stranded RNA viruses, including human flaviviruses and coronaviruses. Phase 2 interim virology analysis reported in June 2021 included data from 62 of 70 hospitalized patients with moderate COVID-19 symptoms who received the drug or placebo BID for 5 days. On Day 2 of treatment, patients taking bemnifosbuvir had an 80% (0.7 log10) greater mean reduction from baseline viral load compared with placebo. No detectable levels of virus were observed at 2 weeks in 47% of the bemnifosbuvir group compared with 22% in the placebo group. [197]
Additional global trials for bemnifosbuvir include a phase 3 trial (MORNINGSKY) in outpatients with mild-to-moderate COVID-19 disease. The trial will evaluate bemnifosbuvir as both monotherapy and combination antiviral therapy in outpatients with COVID-19 who are at high risk for disease progression. [198] Another phase 3 trial (MEADOWSPRING) is being conducted as a 6-month long-term follow-on study to evaluate the impact of prior administration of bemnifosbuvir on long COVID in patients previously enrolled in MORNINGSKY.
Clinical trials of existing drugs with potential antiviral properties
Nitazoxanide
Nitazoxanide, a broad-spectrum thiazolide antiparasitic agent, is approved in the United States for treatment of Cryptosporidium parvum and Giardia duodenalis infections. The NIH recommends against use of nitazoxanide for treatment of COVID-19, except in a clinical trial. A systematic review of 5 blinded, placebo-controlled randomized clinical trials showed no evidence of clinical benefits to treat patients with mild or moderate COVID-19. A reduction in WBC, LDH, and D-dimer levels among nitazoxanide-treated patients was observed, but the effect size was considered small to moderate. [199]
Niclosamide
Niclosamide (FW-1002 [FirstWave Bio]; ANA001 [ ANA Therapeutics]) is an anthelmintic agent used primarily for tapeworms for nearly 50 years. Niclosamide is thought to disrupt SARS-CoV-2 replication through S-phase kinase-associated protein 2 (SKP2)-inhibition, by preventing autophagy and blocking endocytosis.
A proprietary formulation that targets the viral reservoir in the gut to decrease prolonged infection and transmission has been developed, specifically to decrease gut viral load. It is being tested in a phase 2 trial. [200] A phase 2/3 trial is testing safety and the potential to improved outcomes and reduce hospital stay by reducing viral load. [201]
Immunomodulators and Other Investigational Therapies
Early in the pandemic, drugs (eg, interleukin inhibitors, Janus kinase inhibitor) were identified that may modulate the immunologic pathways associated with the hyperinflammation observed with COVID-19. [202, 203] Since then, several have been approved by the FDA (ie, baricitinib) or have been granted emergency use authorization (ie, tocilizumab, anakinra).
A study comparing baricitinib and tocilizumab found no difference in mortality between the 2 treatments. Occurrence of adverse effects was higher in the tocilizumab treated patients compared with baricitinib, including secondary infections secondary infections (32% vs 22%; p < 0.01); thrombotic events (24% vs 16%; p < 0.01); and acute liver injury (8% vs 3%; p < 0.01). [204, 205]
Janus Kinase Inhibitors
Drugs that target numb-associated kinase (NAK) may mitigate systemic and alveolar inflammation in patients with COVID-19 pneumonia by inhibiting essential cytokine signaling involved in immune-mediated inflammatory response. In particular, NAK inhibition has been shown to reduce viral infection in vitro. ACE2 receptors are a point of cellular entry by COVID-19, which is then expressed in lung AT2 alveolar epithelial cells. A known regulator of endocytosis is the AP2-associated protein kinase-1 (AAK1). The ability to disrupt AAK1 may interrupt intracellular entry of the virus. Baricitinib (Olumiant; Eli Lilly Co), a Janus kinase (JAK) inhibitor, is also identified as a NAK inhibitor with a particularly high affinity for AAK1. [206, 207, 208]
Baricitinib
Baricitinib is the first immunotherapy to gain full FDA approval in May 2022 for treatment of hospitalized adults who require supplemental oxygen, noninvasive or invasive mechanical ventilation, or extracorporeal membrane oxygenation (ECMO). Approval was based on the ACTT-2 and COV-BARRIER trials.
Emergency use authorization (EUA) was issued by the FDA for baricitinib on November 19, 2020, and remains in place for children aged 2-17 years following approval for adults.
The NIAID Adaptive Covid-19 Treatment Trial (ACTT-2) evaluated the combination of baricitinib (4 mg PO daily up to 14 days) and remdesivir (100 mg IV daily up to 10 days) (515 patients) compared with remdesivir plus placebo (518 patients). Patients who received baricitinib had a median time to recovery of 7 days compared with 8 days with control (P = 0.03), and a 30% higher odds of improvement in clinical status at Day 15. Those receiving high-flow oxygen or noninvasive ventilation at enrollment had a time to recovery of 10 days with combination treatment and 18 days with control (rate ratio for recovery, 1.51). The 28-day mortality was 5.1% in the combination group and 7.8% in the control group (hazard ratio for death, 0.65). Incidence of serious adverse events were less frequent in the combination group than in the control group (16.0% vs 21.0%; P = 0.03) There were also fewer new infections in patients who received baricitinib (5.9% vs 11.2%; P =0 .003). [209]
The COV-BARRIER trial demonstrated baricitinib to be the first immunomodulatory treatment to reduce COVID-19 mortality in a placebo-controlled trial. [210] Results from the global COV-BARRIER phase 3 trial showed a reduced risk for death in hospitalized patients not on mechanical ventilation who received baricitinib 4 mg daily for up to 14 days when added to standard of care (SOC), compared with SOC alone at Day 28 (38.2% risk reduction in mortality; (62/764 [8.1%] baricitinib; 101/761 [13.3%] placebo; p = 0.0018). Progression to high-flow oxygen, noninvasive ventilation, or invasive mechanical ventilation did not reach statistical significance for baricitinib plus SOC compared with SOC alone (27.8% vs 30.5%; p = 0.0018). The 60-day all-cause mortality was 10% (n=79) for baricitinib and 15% (n=116) for placebo (p = 0.005). Serious adverse events occurred in 15% of the baricitinib group compared with 18% of those receiving placebo. Serious infections (9% vs 10%) and venous thromboembolic events (3% in each group) were similar between the 2 groups. [211]
The COV-BARRIER study was expanded to include patients on mechanical ventilation. Those who received baricitinib plus SOC and on mechanical ventilation or ECMO were 46% less likely to die by Day 28 compared with patients on SOC alone (p = 0.0296). The cumulative proportion among these patients who died by Day 28 was 39.2% (20/51) in the baricitinib arm compared with 58% in the placebo arm (29/50). [212]
Tofacitinib
Tofacitinib (Xeljanz), another JAK inhibitor, was evaluated in 289 hospitalized patients with COVID-19 pneumonia who were randomized 1:1 at 15 sites in Brazil. Most patients (89.3%) received glucocorticoids during hospitalization. Cumulative incidence of death or respiratory failure through Day 28 was 18.1% in the tofacitinib group and 29% in the placebo group (P = 0.04). Death from any cause through Day 28 occurred in 2.8% of the patients in the tofacitinib group and in 5.5% of those in the placebo group. [213]
Interleukin Inhibitors
Interleukin (IL) inhibitors may ameliorate severe damage to lung tissue caused by cytokine release in patients with serious COVID-19 infections. Several studies have indicated a “cytokine storm” with release of IL-6, IL-1, IL-12, and IL-18, along with tumor necrosis factor alpha (TNFα) and other inflammatory mediators. The increased pulmonary inflammatory response may result in increased alveolar-capillary gas exchange, making oxygenation difficult in patients with severe illness.
Interleukin-6 inhibitors
IL-6 is a pleiotropic proinflammatory cytokine produced by various cell types, including lymphocytes, monocytes, and fibroblasts. SARS-CoV-2 infection induces a dose-dependent production of IL-6 from bronchial epithelial cells. This cascade of events is the rationale for studying IL-6 inhibitors. [214]
Tocilizumab
Tocilizumab was issued an EUA on June 24, 2021 for hospitalized adults and pediatric patients (aged 2 years and older) with COVID-19 who are receiving systemic corticosteroids and require supplemental oxygen, noninvasive or invasive mechanical ventilation, or extracorporeal membrane oxygenation (ECMO). The FDA granted full approval for this indication for adults in December 2022. The EUA remains in place for children.
The Infectious Disease Society of America guidelines recommend tocilizumab in addition to standard of care (ie, steroids) among hospitalized adults with COVID-19 who have elevated markers of systemic inflammation. [26] The NIH guidelines recommend use of tocilizumab (single IV dose of 8 mg/kg, up to 800 mg) in combination with dexamethasone in recently hospitalized patients who are exhibiting rapid respiratory decompensation caused by COVID-19. [215] These recommendations are based on the paucity of evidence from randomized clinical trials to show certainty of mortality reduction.
The EMPACTA trial found nonventilated hospitalized patients who received tocilizumab (n = 249) in the first 2 days of ICU admission had a lower risk for progression to mechanical ventilation or death by day 28 compared with those not treated with tocilizumab (n = 128) (12% vs 19.3%, respectively). The data cutoff for this study was September 30, 2020. In the 7 days before the trial or during the trial, 200 patients in the tocilizumab group (80.3%) and 112 patients in the placebo group (87.5%) received systemic glucocorticoids and 55.4% and 67.2% of the patients received dexamethasone. Antiviral treatment was administered in 196 (78.7%) and 101 (78.9%), respectively, and 52.6% and 58.6% received remdesivir. However, there was no difference in incidence of death from any cause between the 2 groups. [216]
The REMDACTA trial did not show additional benefit for tocilizumab plus remdesivir compared with remdesivir alone in patients with severe COVID-19 pneumonia. Among 649 enrolled patients, 434 were randomly assigned to tocilizumab plus remdesivir and 215 to placebo plus remdesivir. There were 566 patients (88.2%) who also received corticosteroids during the trial to Day 28. Median time from randomization to hospital discharge was 14 days for each group. Also, there was no significant difference in deaths by Day 28 between each treatment group. [217]
Results from the REMAP-CAP international adaptive trial evaluated efficacy of tocilizumab 8 mg/kg (n = 353), sarilumab 400 mg (n = 48), or control (n = 402) in critically ill hospitalized adults receiving organ support in intensive care. Hospital mortality at Day 21 was 28% (98/350) for tocilizumab, 22.2% (10/45) for sarilumab, and 35.8% (142/397) for control. Of note, corticosteroids became part of the standard of care midway through the trial. Estimates of the treatment effect for patients treated with either tocilizumab or sarilumab and corticosteroids in combination were greater than for any single intervention. [218]
The RECOVERY trial assessed use of 4,116 hospitalized adults with COVID-19 infection who received either tocilizumab (n = 2022) compared with standard of care (n = 2094) in the United Kingdom from April 23, 2020 to January 24, 2021. Among participants, 562 (14%) received invasive mechanical ventilation, 1686 (41%) received non-invasive respiratory support, and 1868 (45%) received no respiratory support other than oxygen. Median C-reactive protein was 143 mg/L and most patients (82% in both treatment groups) were receiving systemic corticosteroids at randomization. Tocilizumab mortality benefits were clearly seen among those who also received systemic corticosteroids. Patients in the tocilizumab group were more likely to be discharged from the hospital within 28 days (57% vs 50; P< 0.0001). Among those not receiving invasive mechanical ventilation at baseline, patients who received tocilizumab were less likely to reach the composite endpoint of invasive mechanical ventilation or death (35% vs 42%; P< 0.0001). [219]
Conversely, the COVACTA study, 452 with COVID-19 (oxygen saturation, 93% or less) were randomly assigned in a 2:1 ratio to receive 1 dose of tocilizumab or placebo. At Day 28, no significant difference was observed for mortality between the tocilizumab group and placebo (19.7% vs 19.4%, respectively). [220]
An editorial by Rubin et al discusses the discordant results of the RECOVERY and REMAP-CAP trials compared with the COVACTA trial. One significant difference noted is that patients with severe disease now almost universally receive glucocorticoids. Only a minority of patients in the COVACTA trial were treated with glucocorticoids. Fewer patients reeived glucocorticoids in the tocilizumab group (19.4%) compared with those in the placebo group (28.5%). In contrast, 93% and 82% of all patients in REMAP-CAP and the RECOVERY trial, respectively, were receiving glucocorticoid therapy. [221]
Average wholesale price of tocilizumab is approximately $5000 for an 800-mg dose. Preliminary results for sarilumab have also been reported.
Interleukin-1 inhibitors
Anakinra
Anakinra was issued an EUA on November 8, 2022 for treatment of COVID-19 pneumonia in hospitalized adults on supplemental oxygen (low- or high-flow) who are at risk of progressing to severe respiratory failure and likely to have an elevated plasma soluble urokinase plasminogen activator receptor (suPAR).
Hospitalized patients with COVID-19 at increased risk for respiratory failure showed significant improvement after treatment with anakinra compared with placebo, based on data from a phase 3, randomized, confirmatory trial (SAVE-MORE study; n = 594). Patients in each study arm also received standard of care treatment. Patients were identified by increased suPAR serum levels, which is an early indicator of progressing respiratory failure.
The anakinra-treated group had lower odds of more severe disease at Day 28 compared with placebo. There were 13 deaths (3.2%) in the anakinra arm and 13 deaths (6.9%) in the placebo arm. Also by Day 28, there were 86 patients (21.2%) in the anakinra arm and 62 patients (32.8%) in the placebo arm who developed severe respiratory failure. By Day 60, there were 21 deaths (5.3%) in the anakinra arm and 18 deaths (9.7%) in the placebo arm. [] 211]
Endogenous IL-1 levels are elevated in individuals with COVID-19 and other conditions, such as severe CAR-T-cell–mediated cytokine-release syndrome. Anakinra has been used off-label for this indication. As of June 2020, the NIH guidelines note insufficient data to recommend for or against use of IL-1 inhibitors. [222]
Interleukin-7 inhibitors
The recombinant interleukin-7 inhibitor, CYT107 (RevImmune), increases T-cell production and corrects immune exhaustion. Several phase 2 clinical trials have been completed in France, Belgium, and the UK to assess immune reconstitution in lymphopenic patients with COVID-19. [223, 224, 225] Phase 2 trials were initiated in November 2020 in the United States.
Corticosteroids
The UK RECOVERY trial assessed the mortality rate at Day 28 in hospitalized patients with COVID-19 who received low-dose dexamethasone 6 mg PO or IV daily for 10 days added to usual care. Patients were assigned to receive dexamethasone (n = 2104) plus usual care or usual care alone (n = 4321). Overall, 482 patients (22.9%) in the dexamethasone group and 1110 patients (25.7%) in the usual care group died within 28 days after randomization (P< 0.001). In the dexamethasone group, the incidence of death was lower than in the usual care group among patients receiving invasive mechanical ventilation (29.3% vs 41.4%) and among those receiving oxygen without invasive mechanical ventilation (23.3% vs 26.2%), but not among those who were receiving no respiratory support at randomization (17.8% vs 14%). [25]
Corticosteroids generally are not recommended for treatment of viral pneumonia. [226] The benefit of corticosteroids in septic shock results from tempering the host immune response to bacterial toxin release. The incidence of shock in patients with COVID-19 is relatively low (5% of cases). It is more likely to produce cardiogenic shock from increased work of the heart needed to distribute oxygenated blood supply and thoracic pressure from ventilation. Corticosteroids can induce harm through immunosuppressant effects during the treatment of infection and have failed to provide a benefit in other viral epidemics, such as respiratory syncytial virus (RSV) infection, influenza infection, SARS, and MERS. [227]
Early guidelines for management of critically ill adults with COVID-19 specified when to use low-dose corticosteroids and when to refrain from using corticosteroids. The recommendations depended on the precise clinical situation (eg, refractory shock, mechanically ventilated patients with ARDS); however, these particular recommendations were based on evidence listed as weak. [228] The results from the RECOVERY trial in June 2020 provided evidence for clinicians to consider when low-dose corticosteroids would be beneficial. [25]
Several trials examining use of corticosteroids for COVID-19 were halted after publication of the RECOVERY trial results; however, a prospective meta-analysis from the WHO rapid evidence appraisal for COVID-19 therapies (REACT) pooled data from 7 trials (eg, RECOVERY, REMAP-CAP, CoDEX, CAP COVID) that totaled 1703 patients (678 received corticosteroids and 1025 received usual care or placebo). An association between corticosteroids and reduced mortality was similar for dexamethasone and hydrocortisone, suggesting the benefit is a general class effect of glucocorticoids. The 28-day mortality rate, the primary outcome, was significantly lower among corticosteroid users (32% absolute mortality for corticosteroids vs 40% assumed mortality for controls). [229] An accompanying editorial addresses the unanswered questions regarding these studies. [230]
The WHO guidelines for use of dexamethasone (6 mg IV or oral) or hydrocortisone (50 mg IV every 8 hours) for 7-10 days in the most seriously ill patients coincides with publication of the meta-analysis. [231]
Complement Inhibitors
Poor COVID-19 disease outcomes have been associated with activation of the complement system, specifically the C5a-C5aR axis. [232, 233] Studies have shown C5a attracts neutrophils and monocytes to the infection site, which may lead to tissue damage, endothelialitis, and microthrombosis. [234]
Vilobelimab
Vilobelimab (Gohibic; InflaRx) was granted an EUA by the FDA on April 4, 2023 for treatment of coronavirus disease 2019 (COVID-19) in hospitalized adults when initiated within 48 hr of receiving invasive mechanical ventilation (IMV) or extracorporeal membrane oxygenation (ECMO). It is a chimeric human/mouse immunoglobulin G4 (IgG4) antibody consisting of mouse anti-human complement factor 5a (C5a) monoclonal binding sites.
Evidence from the multicenter, double-blind, randomized, placebo-controlled phase 3 PANAMO trial reported results from 369 patients who were randomly assigned to receive vilobelimab (n =177) or placebo (n = 191). Both groups received standard of care (eg, anticoagulants, dexamethasone, and/or other immunomodulators). The data estimated 28-day mortality rate was 31.7% in the vilobelimab group compared with 41.6% with placebo (p < 0.05), which correlated to a 23.9% risk reduction. [235]
Human Vasoactive Intestinal Polypeptides
Aviptadil (Zyesami; RLF-100; NeuroRx) is a synthetic vasoactive intestinal peptide (VIP) that prevents NMDA-induced caspase-3 activation in lungs and inhibits IL-6 and TNF-alpha production. An EUA was submitted to the FDA on June 1, 2021 to treat critically ill patients with COVID-19 infection and respiratory failure. Results from a phase 2b/3 trial (COVID-AIV) of IV aviptadil for treatment of respiratory failure in critically ill patients with COVID-19 demonstrated meaningful recovery at days 28 (P = 0.014) and 60 (P = 0.013) and survival (P< 0.001). Patients enrolled in the study had respiratory failure despite prior treatment with all approved medicines for COVID-19 including remdesivir. Other therapies administered included steroids, anticoagulants, and various monoclonal antibodies. Analysis of patients who remained in respiratory failure despite treatment with remdesivir identified a statistically significant (P = .03) 2.5-fold increased odds of being alive and free of respiratory failure and a statistically significant (P = .006) fourfold higher odds of being alive at day 60 among patients treated with aviptadil compared with those treated with placebo. Although antiviral treatment has shown advantages in treating patients with earlier stages of COVID-19, aviptadil is the first to demonstrate increased recovery and survival in patients who have already progressed to respiratory failure. [236]
Aviptadil is being studied as part of the NIH’s ACTIV-3 critical care protocol alone and in combination with remdesivir in hospitalized patients with ARDS.
Additionally, it is being studied as an inhaled treatment. [237]
SYK Inhibitors
Fostamatinib (Tavalisse; Rigel Pharmaceuticals) is a spleen tyrosine kinase (SYK) inhibitor that reduces signaling by Fc gamma receptor (FcγR) and c-type lectin receptor (CLR), which are drivers of proinflammatory cytokine release. It also reduces mucin-1 protein abundance, which is a biomarker used to predict ARDS development. It is approved in the United States for thrombocytopenia in patients with chronic immune thrombocytopenia (ITP). The active metabolite (R406) inhibits signal transduction of Fc-activating receptors and B-cell receptor to reduce antibody-mediated destruction of platelets.
The phase 2 NIH trial randomly assigned 59 hospitalized patients (30 to fostamatinib and 29 to placebo) with COVID-19 in addition to standard of care. There were 3 deaths that occurred by Day 29, all receiving placebo. The mean change in ordinal score at Day 15 was greater in the fostamatinib group (-3.6 ± 0.3 vs. -2.6 ± 0.4; P = .035) and the median length in the ICU was 3 days in the fostamatinib group compared with 7 days in placebo (P = .07). Differences in clinical improvement were most evident in patients with severe or critical disease (median days on oxygen, 10 vs. 28; P = .027). [238]
Interferons
Interferon lambda
Receptors for lambda-interferon are generally located in the lining of the lungs, airways, and intestine – the locations where SARS-CoV-2 is often introduced. An international study in Canada and Brazil showed efficacy of a single subcutaneous injection of peglylated interferon lambda in outpatients significantly reduced the incidence of hospitalization or an emergency room visit (for < 6 hours) compared with those who received placebo. Nonhospitalized patients were administered 180 mg SC of pegylated interferon lambda (n = 933) or placebo (n = 1018). The effects were consistent across dominant variants and independent of vaccination status. [239]
Interferon beta-1a
Interferon is a natural antiviral part of the immune system. Interferon impairment is associated with the pathogenesis and severity of COVID-19 infection. The NIAID’s Adaptive COVID-19 Treatment Trial (ACTT-3) compared SC interferon beta-1a (Rebif) plus remdesivir (n = 487) with remdesivir plus placebo (n = 482) in hospitalized patients. Results showed interferon beta-1a plus remdesivir was not superior to remdesivir alone. Additionally, in patients who required high-flow oxygen at baseline, adverse effects were higher among those receiving remdesivir plus interferon beta-1a group compared with remdesivir plus placebo. (69% vs 39%). Serious adverse events in the interferon beta-1a plus remdesivir group were also higher compared with remdesivir alone (60% vs 24%). [240]
Miscellaneous Therapies
Nitric Oxide
The Society of Critical Care Medicine recommends against the routine use of iNO in patients with COVID-19 pneumonia. Instead, they suggest a trial only in mechanically ventilated patients with severe ARDS and hypoxemia despite other rescue strategies. [228] The cost of iNO is reported as exceeding $100/hour.
Statins
In addition to the cholesterol-lowering abilities of HMG-CoA reductase inhibitors (statins), they also decrease the inflammatory processes of atherosclerosis. [241] Because of this, questions have arisen whether statins may be beneficial to reduce inflammation associated with COVID-19. RCTs of statins as anti-inflammatory agents for viral infections are limited, and results have been mixed.
Two meta-analyses have shown opposing conclusions regarding outcomes of patients who were taking statins at the time of COVID-19 diagnosis. [242, 243] Randomized controlled trials are needed to examine the ability of statins to attenuate inflammation, presumably by inhibiting expression of the MYD88 gene, which is known to trigger inflammatory pathways. [244]
Adjunctive Nutritional Therapies
NIH guidelines state there is insufficient evidence to recommend either for or against use of vitamins C and D, and zinc for treatment of COVID-19. The guidelines recommend against using zinc supplementation above the recommended dietary allowance.
Vitamin and mineral supplements have been promoted for the treatment and prevention of respiratory viral infections; however, there is insufficient evidence to suggest a therapeutic role in treating COVID-19. [245]
Zinc
A retrospective analysis showed lack of a causal association between zinc and survival in hospitalized patients with COVID-19. [246]
Vitamin D
A study found individuals with untreated vitamin D deficiency were nearly twice as likely to test positive for COVID-19 compared with peers with adequate vitamin D levels. Among 489 individuals, vitamin D status was categorized as likely deficient for 124 participants (25%), likely sufficient for 287 (59%), and uncertain for 78 (16%). Seventy-one participants (15%) tested positive for COVID-19. In a multivariate analysis, a positive COVID-19 test was significantly more likely in those with likely vitamin D deficiency than in those with likely sufficient vitamin D levels (relative risk [RR], 1.77; P = .02). Testing positive for COVID-19 was also associated with increasing age up to age 50 years (RR, 1.06; P = .02) and race other than White (RR, 2.54; P = .009). [247] It is unknown if vitamin D deficiency is the specific issue, as it also is associated with various conditions that are risk factors for severe COVID-19 conditions (eg, advanced age, cardiovascular disease, diabetes mellitus). [248]
Extended-release formulation of calcifediol (25-hydroxyvitamin D3 [Rayaldee; OPKO Health]), a prohormone of the active form of vitamin D3. Phase 2 (REsCue) completed. The objective was to raise and maintain serum total 25-hydroxyvitamin D levels to at least 25 ng/mL to mitigate COVID-19 severity in outpatients (average age 43 y; range 18-71 y). Preliminary data suggest earlier resolution of chest congestion in patients treated with 4 weeks of calcifediol compared with placebo. [249]
Investigational Antibody-Directed Therapies
COVID-19 Convalescent Plasma
The FDA granted emergency use authorization (EUA) on August 23, 2020 for use of COVID-19 convalescent plasma (CCP) in hospitalized patients. Convalescent plasma contains antibody-rich plasma products collected from eligible donors who have recovered from COVID-19. The EUA limits the authorization to use of CCP products that contain high levels of anti-SARS-CoV-2 antibodies for treatment of outpatients or inpatients with COVID-19 who have immunosuppressive disease or who are receiving immunosuppressive treatment. [250]
As of January 2023, high-titer CCP once again has become important to immunosuppressed patients since the EUAs for monoclonal antibodies have been revoked owing to SARS-CoV-2 variants that are no longer susceptible. A systematic review and meta-analysis concluded that transfusion of CCP is associated with decreasing mortality among patients who are immunocompromised and have COVID-19. [251]
The REMAP-CAP investigators concluded that among critically ill adults with confirmed COVID-19, treatment with 2 units of high-titer, ABO-compatible convalescent plasma had a low likelihood of providing improvement in the number of organ support–free days. The study’s primary end point was organ support–free days (days alive and free of intensive care unit–based organ support) up to day 21. Among 2011 participants who were randomized, 1990 (99%) completed the trial. The convalescent plasma intervention was stopped after the prespecified criterion for futility was met. Median number of organ support–free days was 0 in the convalescent plasma group and 3 in the no convalescent plasma group. The in-hospital mortality rate was 37.3% (401/1075) for the convalescent plasma group and 38.4% (347/904) for the no convalescent plasma group and the median number of days alive and free of organ support was 14 for each group. [252]
Monoclonal Antibodies
No EUAs for SARS-CoV-2 monoclonal antibodies remain active in the United States as of January 26, 2023 owing to high frequency of circulating SARS-CoV-2 variants that are non-susceptible.
Monoclonal Antibodies Whose Distribution is Paused
Distribution of the following monoclonal antibodies has been paused in the United States owing to loss of efficacy to the viral variants.
Vaccines
mRNA vaccine (Comirnaty; Pfizer) and mRNA-1273 (Spikevax; Moderna) have gained full FDA approval. Other SARS-CoV-2 vaccine available in the United States through emergency use authorization include an adjuvanted protein subunit vaccine – NVX-CoV2373 (Novavax) and a viral vector vaccine – Ad26.COV2.S (Johnson & Johnson). Two bivalent vaccines for use as boosters were granted EUAs in August 2022 to include enhance coverage for Omicron BA.4/BA.5 subvariants. The FDA has also authorized the monovalent adjuvanted vaccines from Novavax as a first booster in adults. For full discussion regarding vaccines, see COVID-19 Vaccines.
The genetic sequence of SARS-CoV-2 was published on January 11, 2020. The rapid emergence of research and collaboration among scientists and biopharmaceutical manufacturers followed. Various methods are used for vaccine discovery and manufacturing.
In addition to the complexity of finding the most effective vaccine candidates, the production process is also important for manufacturing the vaccine to the scale needed globally. Other variable that increase complexity of distribution include storage requirements (eg, frozen vs refrigerated) and if more than a single injection is required for optimal immunity. Several technological methods (eg, DNA, RNA, inactivated, viral vector, protein subunit) are available for vaccine development. Vaccine attributes (eg, number of doses, speed of development, scalability) depend on the type of technological method employed. For example, the mRNA vaccine platforms allow for rapid development. [263, 264]
Antithrombotics
COVID-19 is a systemic illness that adversely affects various organ systems. A review of COVID-19 hypercoagulopathy aptly describes both microangiopathy and local thrombus formation, and a systemic coagulation defect leading to large vessel thrombosis and major thromboembolic complications, including pulmonary embolism, in critically ill patients. [265] While sepsis is recognized to activate the coagulation system, the precise mechanism by which COVID-19 inflammation affects coagulopathy is not fully understood. [266]
Several retrospective cohort studies have described use of therapeutic and prophylactic anticoagulant doses in critically ill hospitalized patients with COVID-19. No difference in 28-day mortality was observed for 46 patients empirically treated with therapeutic anticoagulant doses compared with 95 patients who received standard DVT prophylaxis doses, including those with D-dimer levels greater than 2 mcg/mL. In this study, Day 0 was the day of intubation, therefore, they did not evaluate all patients who received empiric therapeutic anticoagulation at the time of diagnosis to see if progression to intubation was improved. [267]
In contrast to the above findings, a retrospective cohort study showed a median 21-day survival for patients requiring mechanical ventilation who received therapeutic anticoagulation compared with 9 days for those who received DVT prophylaxis. [268]
NIH Trial
Guidelines include thrombosis prophylaxis (typically with low-molecular-weight heparin [LMWH]) for hospitalized patients. The NIH ACTIV trial includes an arm (ACTIV-4) for use of antithrombotics in the outpatient (trial closed as of June 2021), inpatient, and convalescent settings.
The three adaptive clinical trials within ACTIV-4 include preventing, treating, and addressing COVID-19-associated coagulopathy (CAC). Additionally, a goal to understand the effects of CAC across patient populations – inpatient, outpatient, and convalescent.
Outpatient trial
For nonhospitalized patients with COVID-19, anticoagulants and antiplatelet therapy should not be initiated for the prevention of VTE or arterial thrombosis unless the patient has other indications for the therapy or is participating in a clinical trial.
The ACTIV-4B was initiated mid-2020 to investigate whether anticoagulants or antithrombotic therapy can reduce life-threatening cardiovascular or pulmonary complications in newly diagnosed patients with COVID-19 who do not require hospital admission. Participants were randomized to take either a placebo, aspirin, or a low or therapeutic dose of apixaban. The outpatient thrombosis prevention study was halted as the researchers concluded that among mildly symptomatic but clinically stable COVID-19 outpatients a week or more since the time of diagnosis, rates of major cardio-pulmonary complications are very low and do not justify preventive anticoagulant or antiplatelet therapy unless otherwise clinically indicated. [269]
Inpatient trial
Investigates an approach aimed at preventing clotting events and improving outcomes in hospitalized patients with COVID-19. Results published in August 2021 found full-dose anticoagulation (ie, therapeutic dose parenteral anticoagulation with SC low-molecular weight heparin [LMWH] or IV unfractionated heparin) reduced the need for organ support in moderately ill hospitalized patients (n = 2,219), but not in critically ill patients (n = 1,098). Additionally, full dose anticoagulation in critically ill patients may cause harm compared with those given usual-care thromboprophylaxis (ie, thromboprophylactic dose anticoagulation according to local practice). Among moderately ill patients, researchers found that the likelihood of full-dose heparin to reduce the need for organ support compared to those who received low-dose heparin was 98.6%. To ensure adequate separation between the study groups the dose of heparin/LMWH used in the usual care arm did not equal more than half of the approved therapeutic dose for that agent for the treatment of venous thromboembolism. These results emphasize the need to stratify patients with different disease severity within clinical trials. [270, 271]
Convalescent trial
Investigates safety and efficacy apixaban administered to patients who have been discharged from the hospital or are convalescing in reducing thrombotic complications (eg, MI, stroke, DVT, PE, death). Patients will be assessed for these complications within 45 days of being hospitalized for moderate and severe COVID-19.
Investigational antithrombotics
AB201
AB201 (ARCA Biopharma) is a recombinant nematode anticoagulant protein c2 (rNAPc2) that specifically inhibits tissue factor (TF)/factor VIIa complex and has anticoagulant, anti-inflammatory, and potential antiviral properties. TF plays a central role in inflammatory response to viral infections. The phase 2b/3 clinical trial (ASPEN-COVID-19) completed enrollment (n = 160). The trial randomized 2 AB201 dosage regimens compared with heparin in hospitalized SARS-CoV-2-positive patients with an elevated D-dimer level. The primary endpoint was change in D-dimer level from baseline to Day 8. The phase 3 trial design is contingent upon phase 2b results. [272]
Renin Angiotensin System Blockade and COVID-19
SARS-CoV-2 is known to utilize angiotensin-converting enzyme 2 (ACE2) receptors for entry into target cells. [273] Data are limited concerning whether to continue or discontinue drugs that inhibit the renin-angiotensin-aldosterone system (RAAS), namely angiotensin-converting enzyme inhibitors (ACEIs) and angiotensin receptor blockers (ARBs).
The first randomized study to compare continuing vs stopping (ACEIs) or ARBs receptor for patients with COVID-19 has shown no difference in key outcomes between the 2 approaches. A similar 30-day mortality rate was observed for patients who continued and those who suspended ACE inhibitor/ARB therapy, at 2.8% and 2.7%, respectively (hazard ratio, 0.97). [274]
The BRACE Corona trial design further explains the 2 hypotheses. [274]
One hypothesis suggests that use of these drugs could be harmful by increasing the expression of ACE2 receptors (which the SARS-CoV-2 virus uses to gain entry into cells), thus potentially enhancing viral binding and viral entry.
The other suggests that ACE inhibitors and ARBs could be protective by reducing production of angiotensin II and enhancing the generation of angiotensin 1-7, which attenuates inflammation and fibrosis and therefore could attenuate lung injury.
Concern arose regarding appropriateness of continuation of ACEIs and ARBs in patients with COVID-19 after early reports noted an association between disease severity and comorbidities such as hypertension, cardiovascular disease, and diabetes, which are often treated with ACEIs and ARBs. The reason for this association remains unclear. [275, 276]
The speculated mechanism for detrimental effect of ACEIs and ARBs is related to ACE2. It was therefore hypothesized that any agent that increases expression of ACE2 could potentially increase susceptibility to severe COVID-19 by improving viral cellular entry; [276] however, physiologically, ACE2 also converts angiotensin 2 to angiotensin 1-7, which leads to vasodilation and may protect against lung injury by lowering angiotensin 2 receptor binding. [275, 277] It is therefore uncertain whether an increased expression of ACE2 receptors would worsen or mitigate the effects of SARS-CoV-2 in human lungs.
Vaduganathan and colleagues note that data in humans are limited, so it is difficult to support or negate the opposing theories regarding RAAS inhibitors. They offer an alternate hypothesis that ACE2 may be beneficial rather than harmful in patients with lung injury. As mentioned, ACE2 acts as a counterregulatory enzyme that degrades angiotensin 2 to angiotensin 1-7. SARS-CoV-2 not only appears to gain initial entry through ACE2 but also down-regulates ACE2 expression, possibly mitigating the counterregulatory effects of ACE2. [278]
There are also conflicting data regarding whether ACEIs and ARBs increase ACE2 levels. Some studies in animals have suggested that ACEIs and ARBs increase expression of ACE2, [279, 280, 281] while other studies have not shown this effect. [282, 283]
As uncertainty remains regarding whether ACEIs and/or ARBs increase ACE2 expression and how this effect may influence outcomes in patients with COVID-19, cardiology societies have largely recommended against initiating or discontinuing these medications based solely on active SARS-CoV-2 infection. [284, 285]
A systematic review and meta-analysis found use of ACEIs or ARBs was not associated with a higher risk for mortality among patients with COVID-19 with hypertension or multiple comorbidities, supporting recommendations of medical societies to continue use of these agents to control underlying conditions. [286]
Diabetes and COVID-19
High plasma glucose levels and diabetes mellitus (DM) are known risk factors for pneumonia. [287, 288] Potential mechanisms that may increase the susceptibility for COVID-19 in patients with DM include the following [289] :
Higher-affinity cellular binding and efficient virus entry
Decreased viral clearance
Diminished T-cell function
Increased susceptibility to hyperinflammation and cytokine storm syndrome
Presence of cardiovascular disease
SARS-CoV-2 is known to utilize angiotensin-converting enzyme 2 (ACE2) receptors for entry into target cells. Insulin administration attenuates ACE2 expression, while hypoglycemic agents (eg, glucagonlike peptide 1 [GLP-1] agonists, thiazolidinediones) up-regulate ACE2. [289] Dipeptidyl peptidase 4 (DPP-4) is highly involved in glucose and insulin metabolism, as well as in immune regulation. This protein was shown to be a functional receptor for Middle East respiratory syndrome coronavirus (MERS-CoV), and protein modeling suggests that it may play a similar role with SARS-CoV-2, the virus responsible for COVID-19. [290]
The relationship between diabetes, coronavirus infections, ACE2, and DPP-4 has been reviewed by Drucker. [288] Important clinical conclusions of the review include the following:
Hospitalization is more common for acute COVID-19 among patients with diabetes and obesity.
Diabetic medications need to be reevaluated upon admission.
Insulin is the glucose-lowering therapy of choice, not DPP-4 inhibitors or GLP-1 receptor agonists, in patients with diabetes who are hospitalized with acute COVID-19.
Therapies Determined Ineffective
Hydroxychloroquine or chloroquine
The EUA for treatment of COVID-19 with hydroxychloroquine or chloroquine was issued by the FDA in March 2020 and subsequently revoked in June 2020 owing to safety concerns and lack of efficacy.
Additionally, the NIH halted the Outcomes Related to COVID-19 treated with hydroxychloroquine among the In-patients with Symptomatic Disease (ORCHID) study on June 20, 2020. After the fourth analysis that included more than 470 participants, the NIH data and safety monitoring board determined that, while there was no harm, the study drug was very unlikely to be beneficial to hospitalized patients with COVID-19.
The NIH COVID-19 Treatment Guidelines recommends against the use of chloroquine or hydroxychloroquine and/or azithromycin for the treatment of COVID-19 in hospitalized patients and in nonhospitalized patients.
Doxycycline
A few case reports and small case series have speculated on a use for doxycycline in COVID-19. Most seem to have been searching for an antibacterial to replace azithromycin for use in combination with hydroxychloroquine. In general, the use of HCQ has been abandoned. The anti-inflammatory effects of doxycycline were also postulated to moderate the cytokine surge of COVID-19 and provide some benefits. However, the data on corticosteroid use has returned, and is convincing and strongly suggests their use. It is unclear that doxycycline would provide further benefits. Finally, concomitant bacterial infection during acute COVID-19 is proving to be rare decreasing the utility of antibacterial drugs. Overall, there does not appear to be a routine role for doxycycline.
Lopinavir/ritonavir
The NIH Panel for COVID-19 Treatment Guidelines recommend against the use of lopinavir/ritonavir or other HIV protease inhibitors, owing to unfavorable pharmacodynamics and because clinical trials have not demonstrated a clinical benefit in patients with COVID-19.
The Infectious Diseases Society of America (IDSA) guidelines recommend against the use of lopinavir/ritonavir. The guidelines also mention the risk for severe cutaneous reactions, QT prolongation, and the potential for drug interactions owing to CYP3A inhibition. [26]
The RECOVERY trial concluded no beneficial effect was observed in hospitalized patients with COVID-19 who were randomized to receive lopinavir/ritonavir (n = 1616) compared with those who received standard care (n = 3424). No significant difference for 28-day mortality was shown. Overall, 374 (23%) patients allocated to lopinavir/ritonavir and 767 (22%) patients allocated to usual care died within 28 days (P = 0.60). No evidence was found for beneficial effects on the risk of progression to mechanical ventilation or length of hospital stay. [291]
The WHO discontinued use of lopinavir/ritonavir in the SOLIDARITY trial in hospitalized patients on July 4, 2020. [146] Interim results released mid-October 2020 found lopinavir/ritonavir (with or without interferon) appeared to have little or no effect on hospitalized patients with COVID-19, as indicated by overall mortality, initiation of ventilation, and duration of hospital stay. Death rate ratios were: lopinavir, 1.00 (P = 0.97; 148/1399 vs 146/1372) and lopinavir plus interferon, 1.16 (P = 0.11; 243/2050 vs 216/2050). [147]
Ivermectin
NIH COVID-19 guidelines for ivermectin provide analysis of several randomized trials and retrospective cohort studies of ivermectin use in patients with COVID-19. The guidelines concluded most of these studies had incomplete information and significant methodological limitations, which make it difficult to exclude common causes of bias. Ivermectin has been shown to inhibit SAR-COV-2 in cell cultures; however, available pharmacokinetic data from clinically relevant and excessive dosing studies indicate that the SARS-CoV-2 inhibitory concentrations for ivermectin are not likely attainable in humans. [292]
Chaccour and colleagues raised concerns regarding ivermectin-associated neurotoxicity, particularly in patients with a hyperinflammatory state possible with COVID-19. In addition, drug interactions with potent CYP3A4 inhibitors (eg, ritonavir) warrant careful consideration of coadministered drugs. Finally, evidence suggests that ivermectin plasma levels with meaningful activity against COVID-19 would not be achieved without potentially toxic increases in ivermectin doses in humans. More data are needed to assess pulmonary tissue levels in humans. [293]
A prospective study (n = 400) of adults with mild COVID-19 were randomized 1:1 to receive ivermectin 300 mcg/kg/day for 5 days or placebo. Ivermectin did not improve time to symptom resolution in patients with mild COVID-19 disease compared with placebo (P = 0.53). [294]
The Ivermectin Treatment Efficacy in COVID-19 High-Risk Patients (I-TECH) study was an open-label randomized clinical trial conducted at 20 public hospitals and a COVID-19 quarantine center in Malaysia between May 31 and October 25, 2021. Ivermectin was initiated within the first week of patients’ symptom onset. The study included patients aged 50 years and older with laboratory-confirmed SARS-CoV-2, comorbidities, and mild-to-moderate disease. Patients were randomized 1:1 to receive oral ivermectin 400 mcg/kg/day for 5 days plus standard of care (n = 241) or standard of care alone (n = 249). Progression to severe disease did not differ between patients who received ivermectin vs those that did not (P = 0.25). Additionally, no significant differences were observed between the 2 groups regarding mechanical ventilation (P = 0.17), intensive care unit admission (P = 0.79), or 28-day in-hospital death (P = 0.09). [295]
A double-blind, randomized, placebo-controlled adaptive trial (TOGETHER) in Brazil found ivermectin did not lower incidence of medical admission to a hospital owing to progression of COVID-19 or of prolonged emergency department observation among outpatients with an early diagnosis of COVID-19. Findings were similar in patients who received at least 1 dose and those with 100% adherence to the assigned regimen. [296]
Results from the ACTIV-6 NIH trial concluded 400 mcg/kg daily for 3 days resulted in less than 1 day of shortening of symptoms and did not lower incidence of hospitalization or death among outpatients with COVID-19 during the delta and omicron variant time periods. [297]
The ACTIV-6 trial investigators also evaluated efficacy of ivermectin at a maximum targeted dose of 600 mcg/kg daily for 6 days among outpatients with early mild-to-moderate COVID-19. The median time to sustained recovery did not differ between the treated (n = 602) and placebo (n = 604) groups (ie, 11 days (range, 11-12 days. The hazard ratio (posterior probability of benefit) for improvement in time to recovery was 1.02 (95% credible interval, 0.92-1.13; P = 0.68). [298]
Finally, the phase 3, double-blind COVID-OUT trial concluded ivermectin did not prevent occurrence of hypoxemia, emergency department visit, hospitalization, or death associated with COVID-19. [299]
Fluvoxamine
In a murine sepsis model, fluvoxamine was found to bind to the sigma-1 receptor on immune cells, resulting in reduced production of inflammatory cytokines. Results from a small double-blind trial were encouraging.
The TOGETHER trial examined a primary outcome of clinical deterioration, defined as shortness of breath or hospitalization for shortness of breath or pneumonia, and oxygen saturation less than 92% on room air or need for supplemental oxygen to achieve oxygen saturation of 92% or greater. Within 15 days, none of the participants who received fluvoxamine and 8.3% of those who received placebo reached the primary endpoint (P = 0.009). Despite these promising results, limitations (ie, low statistical power and missing data for the primary outcome) precluded definitive conclusions regarding the efficacy of fluvoxamine for the treatment of COVID-19. [300]
The phase 3, double-blind COVID-OUT trial concluded fluvoxamine did not prevent occurrence of hypoxemia, emergency department visit, hospitalization, or death associated with COVID-19. [299]
The ACTIV-6 study group compared the efficacy of low-dose (50 mg BID) fluvoxamine (n = 674) for 10 days versus placebo (n = 614) in patients at least 30 years old with SARS-CoV-2 infection who were experiencing 2 or more symptoms of acute COVID-19 for less than 7 days. Median time to sustained recover was 12 and 13 days in the fluvoxamine and placebo groups, respectively. Overall, 3.9 and 3.8% of participants in the fluvoxamine and placebo groups, respectively, had the composite outcome of hospitalization, urgent care visit, or emergency department visit through day 28. One and 2 participants in the fluvoxamine and placebo groups, respectively, were hospitalized. No deaths were reported in either group. Findings did not support fluvoxamine use at this dose for mild-to-moderate COVID-19. [301]
Favipiravir
Favipiravir is an oral antiviral that disrupts viral replication by selectively inhibiting RNA polymerase.A multicenter, randomized, controlled trial (n = 1187) randomized favipiravir to placebo 1:1 and evaluated time to sustained recovery, COVID-19 progression, and cessation of viral shedding. The median time from symptom presentation and from positive test to randomization was 3 and 2 days, respectively. There was no difference between the 2 treatment for any of the endpoints. [302]
QT Prolongation with Potential COVID-19 Pharmacotherapies
Chloroquine, hydroxychloroquine, and azithromycin each carry the warning of QT prolongation and can be associated with an increased risk of cardiac death when used in a broader population. [303] Because of this risk, the American College of Cardiology, American Heart Association, and the Heart Rhythm Society have published a thorough discussion on the arrhythmogenicity of hydroxychloroquine and azithromycin, including a suggested protocol for clinical research QT assessment and monitoring when the two drugs are coadministered. [304, 305]
Giudicessi and colleagues [306] have published guidance for evaluating the torsadogenic potential of chloroquine, hydroxychloroquine, lopinavir/ritonavir, and azithromycin. Chloroquine and hydroxychloroquine block the potassium channel, specifically KCNH2-encoded HERG/Kv11.1. Additional modifiable risk factors (eg, treatment duration, other QT-prolonging drugs, hypocalcemia, hypokalemia, hypomagnesemia) and nonmodifiable risk factors (eg, acute coronary syndrome, renal failure, congenital long QT syndrome, hypoglycemia, female sex, age ≥65 years) for QT prolongation may further increase the risk. Some of the modifiable and nonmodifiable risk factors may be caused by or exacerbated by severe illness.
A cohort study was performed from March 1 through April 7, 2020, to characterize the risk and degree of QT prolongation in patients with COVID-19 who received hydroxychloroquine, with or without azithromycin. Among 90 patients given hydroxychloroquine, 53 received concomitant azithromycin. Seven patients (19%) who received hydroxychloroquine monotherapy developed prolonged QTc of 500 milliseconds or more, and 3 patients (3%) had a change in QTc of 60 milliseconds or more. Of those who received concomitant azithromycin, 11 of 53 (21%) had prolonged QTc of 500 milliseconds or more, and 7 of 53 (13 %) had a change in QTc of 60 milliseconds or more. Clinicians should carefully monitor QTc and concomitant medication usage if considering using hydroxychloroquine. [307]
A retrospective study reviewed 84 consecutive adult patients hospitalized with COVID-19 and treated with hydroxychloroquine plus azithromycin. The QTc increased by greater than 40 ms in 30% of patients. In 11% of patients, QTc increased to more than 500 ms, which is considered a high risk for arrhythmia. The researcher noted that development of acute renal failure, but not baseline QTc, was a strong predictor of extreme QTc prolongation. [308]
A Brazilian study (n=81) compared chloroquine high-dose (600 mg PO BID for 10 days) and low-dose (450 mg BID for 1 day, then 450 mg/day for 4 days). A positive COVID-19 infection was confirmed by RT-PCR in 40 of 81 patients. In addition, all patients received ceftriaxone and azithromycin. Oseltamivir was also prescribed in 89% of patients. Prolonged QT interval (> 500 msec) was observed in 25% of the high-dose group, along with a trend toward higher lethality (17%) compared with lower dose. this prompted the investigators to prematurely halt use of the high-dose treatment arm, noting that azithromycin and oseltamivir can also contribute to prolonged QT interval. The fatality rate was 13.5%. In 14 patients with paired samples, respiratory secretions at day 4 showed negative results in only one patient. [309]
An increased 30-day risk of cardiovascular mortality, chest pain/angina, and heart failure was observed with the addition of azithromycin to hydroxychloroquine. Pooled data from 14 sources of claims data or electronic medical records from Germany, Japan, Netherlands, Spain, United Kingdom, and the United States were analyzed for adverse effects of hydroxychloroquine, sulfasalazine, or the combinations of hydroxychloroquine plus azithromycin or amoxicillin. Overall, 956,374 and 310,350 users of hydroxychloroquine and sulfasalazine, respectively, and 323,122 and 351,956 users of hydroxychloroquine-azithromycin and hydroxychloroquine-amoxicillin, respectively, were included in the analysis. [310]
Investigational Devices Blood purification devices Several extracorporeal blood purification filters (eg, CytoSorb, oXiris, Seraph 100 Microbind, Spectra Optia Apheresis) have received emergency use authorization from the FDA for the treatment of severe COVID-19 pneumonia in patients with respiratory failure. The devices have various purposes, including use in continuous renal replacement therapy or in reduction of proinflammatory cytokines levels. [311] Nanosponges Cellular nanosponges made from plasma membranes derived from human lung epithelial type II cells or human macrophages have been evaluated in vitro. The nanosponges display the same protein receptors required by SARS-CoV-2 for cellular entry and act as decoys to bind the virus. In addition, acute toxicity was evaluated in vivo in mice by intratracheal administration. [312]
Medication sumary
Medication Summary
Remdesivir (an antiviral agent), baricitinib (an immunomodulatory drug), and 2 mRNA vaccines have gained full FDA approval for prevention and treatment of COVID-19 disease.
Investigational treatments include other antiviral agents, vaccines, immunomodulators, convalescent plasma, and antithrombotics. Several of the above therapies have been granted emergency use authorization by the FDA.
As of January 2023, there are no active EUAs for SARS-CoV-2-directed monoclonal antibodies owing to current circulating variants that are non-susceptible.
Treatment does not preclude isolation and masking for those who test positive for SARS-CoV-2.
Vaccines Class Summary The FDA has granted either full approval and/or emergency use authorization for the vaccines listed below. COVID-19 vaccine, mRNA-Pfizer (Comirnaty)
Indicated for active immunization to prevent COVID-19 caused by severe acute respiratory syndrome coronavirus 2 (SARS-CoV-2) in individuals aged 6 months and older. COVID-19 vaccine, mRNA-Moderna (Spikevax)
Indicated for active immunization to prevent COVID-19 caused by severe acute respiratory syndrome coronavirus 2 (SARS-CoV-2) in individuals aged 6 months and older. COVID-19 vaccine, adjuvanted-Novavax (NVX-CoV2373)
Emergency use authorization (EUA) issued by FDA for active immunization to prevent coronavirus disease 2019 (COVID-19) caused by severe acute respiratory syndrome coronavirus 2 (SARS-CoV-2) in individuals aged 12 years and older. COVID-19 bivalent vaccine, mRNA-Pfizer (BNT162b2 OMI)
Omicron BA.4/BA.5-adapted bivalent COVID-19 vaccine is indicated as a single booster dose for adolescents aged 5 years and older. COVID-19 bivalent vaccine, mRNA-Moderna (MRNA-1273.222)
Omicron BA.4/BA.5-adapted bivalent COVID-19 vaccine is indicated as a single booster dose for adolescents aged 5 years and older. COVID-19 vaccine, viral vector-Janssen (Ad26.COV2.S [Johnson & Johnson])
Emergency use authorization (EUA) issued by FDA for active immunization to prevent coronavirus disease 2019 (COVID-19) caused by severe acute respiratory syndrome coronavirus 2 (SARS-CoV-2) in individuals aged 18 years and older.
The EUA was revised in May 2022 to emphasize limited use (ie, for individuals whom other authorized/approved COVID-19 vaccines are not accessible or clinically appropriate, or the individual elects the AD26.COV2.S vaccine and would otherwise not receive a vaccine).
Antiviral Agents Remdesivir was the first antiviral drug approved by the FDA for COVID-19. Two oral antivirals were granted EUAs in December 2021. Remdesivir (Veklury)
Adenosine nucleotide prodrug that distributes into cells, where it is metabolized to form the pharmacologically active nucleoside triphosphate metabolite. Inhibits SARS-CoV-2 RNA-dependent RNA polymerase (RdRp), which is essential for viral replication. It gained full FDA approval for treatment of COVID-19 disease in hospitalized adults and children aged 12 years and older who weigh at least 40 kg. It is also approved as a 3 consecutive day for outpatients aged 12 years and older (weighing at least 40 kg) with mild-to-moderate COVID-19 who are at high risk for progression to severe COVID-19, including hospitalization or death. Also, an EUA has been granted for hospitalized and nonhospitalized pediatric patients weighing 3.5 kg to less than 40 kg or children younger than 12 years who weigh at least 3.5 kg. Nirmatrelvir/ritonavir (Paxlovid)
Oral nirmatrelvir is an inhibitor of SARS-CoV-2 main protease (Mpro), also referred to as 3C-like protease (3CLpro) or nsp5 protease. Inhibition of SARS-CoV-2 Mpro renders it incapable of processing polyprotein precursors, and thereby, preventing viral replication. Nirmatrelvir is boosted with low-dose ritonavir that slows nirmatrelvir metabolism via CYP3A4 inhibition, which results in higher systemic exposure. The EUA is for treatment of mild-to-moderate COVID-19 in adults and pediatric patients aged 12 years and older who weigh at least 40 kg and are at high risk for progression to severe COVID-19, including hospitalization or death. Molnupiravir
Orally bioavailable prodrug of nucleoside analogue beta-D-N4-hydroxycytidine (NHC), which distributes into cells where NHC is phosphorylated to form the pharmacologically active ribonucleoside triphosphate (NHC-TP). Incorporation of NHC-TP into SARS-CoV-2 RNA by viral RNA polymerase results in an accumulation of errors in the viral genome, leading to inhibition of replication. The EUA is for treatment of mild-to-moderate COVID-19 in adults aged 18 years and older and are at high risk for progression to severe COVID-19, including hospitalization or death.
Corticosteroids NIH guidelines for COVID-19 recommends use of dexamethasone to reduce mortality in hospitalized patients who are mechanically ventilated or those requiring supplemental oxygen without mechanical ventilation. [317] These recommendations are based on results of the RECOVERY trial. [25] If dexamethasone is unavailable, use alternant glucocorticoids (eg, prednisone, methylprednisolone, or hydrocortisone). [317] Dexamethasone
Decreases inflammation by suppressing migration of polymorphonuclear leukocytes (PMNs) and reducing capillary permeability; stabilizes cell and lysosomal membranes. Prednisone (Deltasone)
Consider use if dexamethasone is unavailable. Available as oral formulation. Methylprednisolone (A-Methapred, DepoMedrol, Medrol)
Consider use if dexamethasone is unavailable. Available as IV formulation. Hydrocortisone
Consider use if dexamethasone is unavailable. Available as oral or IV formulations.
Immunomodulators Immunomodulators plus other treatment modalities (eg, remdesivir, glucocorticoids) may be considered for hospitalized patients with severe COVID-19 to blunt the hyperinflammation caused by cytokine release. Baricitinib (Olumiant) Baricitinib is the first immunotherapy to gain full FDA approval in May 2022 for treatment of hospitalized adults who require supplemental oxygen, noninvasive or invasive mechanical ventilation, or extracorporeal membrane oxygenation (ECMO). An Emergency use authorization (EUA) was issued by the FDA for baricitinib on November 19, 2020, and remains in place for children aged 2-17 years following approval for adults. Tocilizumab (Actemra)
June 24, 2021: Emergency use authorization (EUA) issued by the FDA for treatment of coronavirus disease 2019 (COVID-19) in hospitalized adults and pediatric patients (aged >2 years) who are receiving systemic corticosteroids and require supplemental oxygen, noninvasive or invasive mechanical ventilation, or ECMO. Anakinra (Kineret)
November 8, 2022 : Emergency use authorization (EUA) granted for treatment of COVID-19 pneumonia in hospitalized adults on supplemental oxygen (low- or high-flow) who are at risk of progressing to severe respiratory failure and likely to have an elevated plasma soluble urokinase plasminogen activator receptor (suPAR).
Complement Inhibitors
Vilobelimab is a chimeric human/mouse immunoglobulin G4 (IgG4) antibody consisting of mouse anti-human complement factor 5a (C5a) monoclonal binding sites. C5a is part of the complement system and is activated as part of the innate immune response initiating an inflammatory cascade that includes increased vascular permeability, coagulation, proinflammatory cytokine release, and recruitment and activation of neutrophils and other myeloid cells.
Vilobelimab (Gohibic)
April 4, 2023: Emergency use authorization (EUA) granted by the FDA for treatment of coronavirus disease 2019 (COVID-19) in hospitalized adults when initiated within 48 hr of receiving invasive mechanical ventilation (IMV) or extracorporeal membrane oxygenation (ECMO).
Monoclonal Antibodies Class Summary Recombinant neutralizing human IgG monoclonal antibodies (mAb) exert their effect by binding to various sites on the SARS-CoV-2 spike protein. Owing to the increase in variants of concern (VOC) in the United States, monoclonal antibodies that have gained emergency use authorization are continually tested to evaluate activity against VOCs. Information, including allocation, for monoclonal antibody treatments for COVID-19 granted emergency use authorization is located at the United States HHS Preparedness and Response. As of January 2023, there are no active EUAs for SARS-CoV-2 directed monoclonal antibodies owing to current circulating variants that are non-susceptible. Tixagevimab and cilgavimab (Evusheld) January 26, 2023: Not currently authorized in any US region owing to high frequency of circulating SARS-CoV-2 variants that are non-susceptible. EUA granted for preexposure prophylaxis of COVID-19 individuals aged 12 years and older (weighing at least 40 kg) who are moderately-to-severely immunocompromised owing to a medical condition or medications/treatment and may no mount an adequate immune response to COVID-19 vaccination, or have a history of severe adverse reactions to a COVID-19 vaccine and/or component(s). Tixagevimab and cilgavimab are long-acting recombinant human IgG1kappa monoclonal antibodies. The antibodies bind to the SARS-CoV-2 virus spike protein, thereby blocking its interaction with the human ACE2 receptor, which is required for virus attachment. Bebtelovimab
November 30, 2022: Not currently authorized in any US region owing to high frequency of circulating SARS-CoV-2 variants that are non-susceptible. EUA granted February 11, 2022. It binds to the spike protein of SARS-CoV-2 and is unmodified in the Fc region. The EUA was granted for treatment of mild-to-moderate coronavirus disease 2019 (COVID-19) in adults and pediatric patients aged 12 years and older (weighing at least 40 kg) who test positive for SARS-CoV-2 and are at high risk for progression to severe COVID-19, including hospitalization or death. Sotrovimab
March/April 2022: Distribution paused owing to low efficacy against omicron BA.2 VOC. FDA granted EUA May 26, 2021. Binds to conserved epitope of the spiked protein of SARS-CoV-1 and SARS-CoV-2, thereby indicating unlikelihood of mutational escape. The EUA was granted for treatment of mild-to-moderate coronavirus disease 2019 (COVID-19) in adults and pediatric patients aged 12 years and older (weighing at least 40 kg) who test positive for SARS-CoV-2 and are at high risk for progression to severe COVID-19, including hospitalization or death. Casirivimab/imdevimab (REGEN-COV)
December 2021: Distribution paused owing to low efficacy against omicron VOC. FDA granted EUA November 21, 2020. Casirivimab and imdevimab IV solution are each supplied in individual single-dose vials and are admixed in the same IV bag. May also be administered SC when an IV infusion is not feasible. In July 2021, the EUA updated to include use as postexposure prophylaxis in additional to treatment for individuals aged 12 years and older (weighing at least 40 kg) at high risk of progression to severe COVID-19, including hospitalization or death, and are not fully vaccinated or are not expected to mount an adequate immune response. Bamlanivimab and etesevimab
December 2021: Distribution paused owing to low efficacy against omicron VOC. EUA for treatment of treatment or postexposure prophylaxis of mild-to-moderate coronavirus disease 2019 (COVID-19) in adults and pediatric patients, including neonates, with positive results of direct severe acute respiratory syndrome coronavirus 2 (SARS-CoV-2) viral testing, and who are at high risk for progressing to severe COVID-19, including hospitalization or death. Etesevimab and bamlanivimab are prepared by admixing each dose within the same IV bag. Etesevimab and bamlanivimab bind to different but overlapping epitopes in the receptor-binding domain of the S-protein. In clinical trials, bamlanivimab and etesevimab administered together resulted in fewer treatment-emergent variants relative to bamlanivimab administered alone.
Comments