Coronavirus Disease 2019 (COVID-19) Part 1
- Izaskun Telliu
- 25 may 2021
- 34 Min. de lectura
Overview
Practice Essentials
Coronavirus disease 2019 (COVID-19) is defined as illness caused by a novel coronavirus called severe acute respiratory syndrome coronavirus 2 (SARS-CoV-2; formerly called 2019-nCoV), which was first identified amid an outbreak of respiratory illness cases in Wuhan City, Hubei Province, China. [1] It was initially reported to the World Health Organization (WHO) on December 31, 2019. On January 30, 2020, the WHO declared the COVID-19 outbreak a global health emergency. [2, 3] On March 11, 2020, the WHO declared COVID-19 a global pandemic, its first such designation since declaring H1N1 influenza a pandemic in 2009. [4]
Illness caused by SARS-CoV-2 was termed COVID-19 by the WHO, the acronym derived from "coronavirus disease 2019." The name was chosen to avoid stigmatizing the virus's origins in terms of populations, geography, or animal associations. [5, 6] On February 11, 2020, the Coronavirus Study Group of the International Committee on Taxonomy of Viruses issued a statement announcing an official designation for the novel virus: severe acute respiratory syndrome coronavirus 2 (SARS-CoV-2). [7]
The Centers for Disease Control and Prevention (CDC) has estimated that SARS-CoV-2 entered the United States in late January or early February 2020, establishing low-level community spread before being noticed. [8] Since that time, the United States has experienced widespread infections, with over 32.6 million reported cases and over 581,000 deaths reported as of May 14, 2021.
On April 3, 2020, the CDC issued a recommendation that the general public, even those without symptoms, should begin wearing face coverings in public settings where social-distancing measures are difficult to maintain to abate the spread of COVID-19. [9]
The CDC had postulated that this situation could result in large numbers of patients requiring medical care concurrently, resulting in overloaded public health and healthcare systems and, potentially, elevated rates of hospitalizations and deaths. The CDC advised that nonpharmaceutical interventions (NPIs) are the most important response strategy for delaying viral spread and reducing disease impact. Unfortunately, these concerns have been proven accurate.
The feasibility and implications suppression and mitigation strategies have been rigorously analyzed and are being encouraged or enforced by many governments to slow or halt viral transmission. Population-wide social distancing plus other interventions (eg, home self-isolation, school and business closures) are strongly advised. These policies may be required for long periods to avoid rebound viral transmission. [10] As the United States is experiencing another surge of COVID-19 infections, the CDC has intensified their recommendations for transmission mitigation. They have recommended universal face mask use, physical distancing, avoiding nonessential indoor spaces, postponing travel, enhanced ventilation, and hand hygiene. [11]
According to the CDC, individuals at high risk for infection include persons in areas with ongoing local transmission, healthcare workers caring for patients with COVID-19, close contacts of infected persons, and travelers returning from locations where local spread has been reported.
The CDC has published a summary of evidence of comorbidities that are supported by meta-analysis/systematic review that have a significant association with risk of severe COVID-19 illness. These include the following conditions [12] :
Cancer
Cerebrovascular disease
Chronic kidney disease
COPD (chronic obstructive pulmonary disease)
Diabetes mellitus, type 1 and type 2
Heart conditions (eg, heart failure, coronary artery disease, cardiomyopathies)
Immunocompromised state from solid organ transplant
Obesity (BMI 30 kg/m 2 or greater)
Pregnancy
Smoking, current or former
Comorbidities that are supported by mostly observational (eg, cohort, case-control, or cross-sectional) studies include [12] :
Children with certain underlying conditions
Down syndrome
HIV (human immunodeficiency virus)
Neurologic conditions, including dementia
Overweight (BMI 25 to less than 30 kg/m 2)
Other lung disease (including interstitial lung disease, pulmonary fibrosis, pulmonary hypertension)
Sickle cell disease
Solid organ or blood stem cell transplantation
Substance use disorders
Use of corticosteroids or other immunosuppressive medications
Comorbidities that are supported by mostly case series, case reports, or, if other study design, the sample size is small include [12] :
Cystic fibrosis
Thalassemia
Comorbidities supported by mixed evidence include [12] :
Asthma
Hypertension
Immune deficiencies
Liver disease
Such individuals should consider the following precautions [12] :
Stock up on supplies.
Avoid close contact with sick people.
Wash hands often.
Stay home as much as possible in locations where COVID-19 is spreading.
Develop a plan in case of illness.
Signs and symptoms
Presentations of COVID-19 range from asymptomatic/mild symptoms to severe illness and mortality. Symptoms may develop 2 days to 2 weeks after exposure to the virus. [13] A pooled analysis of 181 confirmed cases of COVID-19 outside Wuhan, China, found the mean incubation period to be 5.1 days and that 97.5% of individuals who developed symptoms did so within 11.5 days of infection. [14]
Wu and McGoogan reported that, among 72,314 COVID-19 cases reported to the Chinese Center for disease Control and Prevention (CCDC), 81% were mild (absent or mild pneumonia), 14% were severe (hypoxia, dyspnea, >50% lung involvement within 24-48 hours), 5% were critical (shock, respiratory failure, multiorgan dysfunction), and 2.3% were fatal. Multiple reports from around the globe have subsequently confirmed these patterns of presentation. [15]
The following symptoms may indicate COVID-19 [16] :
Fever or chills
Cough
Shortness of breath or difficulty breathing
Fatigue
Muscle or body aches
Headache
New loss of taste or smell
Sore throat
Congestion or runny nose
Nausea or vomiting
Diarrhea
Other reported symptoms have included the following:
Sputum production
Malaise
Respiratory distress
Neurologic (eg, headache, altered mentality)
The most common serious manifestation of COVID-19 appears to be pneumonia.
A complete or partial loss of the sense of smell (anosmia) has been reported as a potential history finding in patients eventually diagnosed with COVID-19. [17] A phone survey of outpatients with mildly symptomatic COVID-19 found that 64.4% (130 of 202) reported any altered sense of smell or taste. [18]
Diagnosis
COVID-19 should be considered a possibility in (1) patients with respiratory tract symptoms and newly onset fever or (2) in patients with severe lower respiratory tract symptoms with no clear cause. Suspicion is increased if such patients have been in an area with community transmission of SARS-CoV-2 or have been in close contact with an individual with confirmed or suspected COVID-19 in the preceding 14 days.
Microbiologic (PCR or antigen) testing is required for definitive diagnosis.
Patients who do not require emergency care are encouraged to contact their healthcare provider over the phone. Patients with suspected COVID-19 who present to a healthcare facility should prompt infection-control measures. They should be evaluated in a private room with the door closed (an airborne infection isolation room is ideal) and asked to wear a surgical mask. All other standard contact and airborne precautions should be observed, and treating healthcare personnel should wear eye protection. [19]
Management
Utilization of programs established by the FDA to allow clinicians to gain access to investigational therapies during the pandemic has been essential. The expanded access (EA) and emergency use authorization (EUA) programs allowed for rapid deployment of potential therapies for investigation and investigational therapies with emerging evidence. A review by Rizk et al describes the role for each of these measures and their importance to providing medical countermeasures in the event of infectious disease and other threats. [20]
On October 22, 2020, remdesivir, an antiviral agent, was the first drug approved for treatment of COVID-19. It is indicated for treatment of COVID-19 disease in hospitalized adults and children aged 12 years and older who weigh at least 40 kg. [21] An emergency use authorization (EUA) remains in place to treat children younger than 12 years who weigh at least 3.5 kg. [22]
An EUA for convalescent plasma was announced on August 23, 2020 and reissued November 30, 2020. [23] Subsequently, the FDA issued guidance to limit use to high titer plasma only.
EUAs have also been granted for outpatient monoclonal directed therapies (ie, casirivimab plus imdevimab, bamlanivimab plus etesevimab) for individuals who test positive and are at high risk of severe COVID-19 or hospitalization.
Baricitinib was issued an EUA on November 19, 2020 for use, in combination with remdesivir, for treatment of suspected or laboratory confirmed coronavirus disease 2019 (COVID-19) in hospitalized patients aged 2 years and older who require supplemental oxygen, invasive mechanical ventilation, or extracorporeal membrane oxygenation (ECMO). [24]
The FDA has granted EUAs for 3 SARS-CoV-2 vaccines since December, 2020. Two are mRNA vaccines – BNT-162b2 (Pfizer) and mRNA-1273 (Moderna), whereas the third is a viral vector vaccine – Ad26.COV2.S (Johnson & Johnson).
Infected patients should receive supportive care to help alleviate symptoms. Vital organ function should be supported in severe cases. [25]
Numerous collaborative efforts to discover and evaluate effectiveness of antivirals, immunotherapies, monoclonal antibodies, and vaccines have rapidly emerged. Guidelines and reviews of pharmacotherapy for COVID-19 have been published. [26, 27, 28, 29, 30]
Background
Coronaviruses comprise a vast family of viruses, 7 of which are known to cause disease in humans. Some coronaviruses that typically infect animals have been known to evolve to infect humans. SARS-CoV-2 is likely one such virus, postulated to have originated in a large animal and seafood market.
Severe acute respiratory syndrome (SARS) and Middle East respiratory syndrome (MERS) are also caused by coronaviruses that “jumped” from animals to humans. More than 8,000 individuals developed SARS, nearly 800 of whom died of the illness (mortality rate of approximately 10%), before it was controlled in 2003. [31] MERS continues to resurface in sporadic cases. A total of 2,465 laboratory-confirmed cases of MERS have been reported since 2012, resulting in 850 deaths (mortality rate of 34.5%). [32]
Route of Transmission
The principal mode by which people are infected with SARS-CoV-2 is through exposure to respiratory droplets carrying infectious virus (generally within a space of 6 feet). Additional methods include contact transmission (eg, shaking hands) and airborne transmission of droplets that linger in the air over long distances (usually greater than 6 feet). [33, 34, 35] Virus released in respiratory secretions (eg, during coughing, sneezing, talking) can infect other individuals via contact with mucous membranes.
On July 9, 2020, the World Health Organization issued an update stating that airborne transmission may play a role in the spread of COVID-19, particularly involving “super spreader” events in confined spaces such as bars, although they stressed a lack of such evidence in medical settings. Thus, they emphasized the importance of social distancing and masks in prevention. [35]
The virus can also persist on surfaces to varying durations and degrees of infectivity, although this is not believed to be the main route of transmission. [33] One study found that SARS-CoV-2 remained detectable for up to 72 hours on some surfaces despite decreasing infectivity over time. Notably, the study reported that no viable SARS-CoV-2 was measured after 4 hours on copper or after 24 hours on cardboard. [36]
In a separate study, Chin and colleagues found that the virus was very susceptible to high heat (70°C). At room temperature and moderate (65%) humidity, no infectious virus could be recovered from printing and tissue papers after a 3-hour incubation period or from wood and cloth by day two. On treated smooth surfaces, infectious virus became undetectable from glass by day 4 and from stainless steel and plastic by day 7. “Strikingly, a detectable level of infectious virus could still be present on the outer layer of a surgical mask on day 7 (~0.1% of the original inoculum).” [37] Contact with fomites is thought to be less significant than person-to-person spread as a means of transmission. [33]
Viral shedding
The duration of viral shedding varies significantly and may depend on severity. Among 137 survivors of COVID-19, viral shedding based on testing of oropharyngeal samples ranged from 8-37 days, with a median of 20 days. [38] A different study found that repeated viral RNA tests using nasopharyngeal swabs were negative in 90% of cases among 21 patients with mild illness, whereas results were positive for longer durations in patients with severe COVID-19. [39] In an evaluation of patients recovering from severe COVID-19, Zhou and colleagues found a median shedding duration of 31 days (range, 18-48 days). [40] These studies have all used PCR detection as a proxy for viral shedding. The Korean CDC, investigating a cohort of patients who had prolonged PCR positivity, determined that infectious virus was not present. [41] These findings were incorporated into the CDC guidance on the duration of isolation following COVID-19 infection.
Additionally, patients with profound immunosuppression (eg, following hematopoietic stem-cell transplantation, receiving cellular therapies) may shed viable SARS-CoV-2 for at least 2 months. [42, 43]
SARS-CoV-2 has been found in the semen of men with acute infection, as well as in some male patients who have recovered. [44]
Asymptomatic/presymptomatic SARS-CoV-2 infection and its role in transmission
Oran and Topol published a narrative review of multiple studies on asymptomatic SARS-CoV-2 infection. Such studies and news articles reported rates of asymptomatic infection in several worldwide cohorts, including resident populations from Iceland and Italy, passengers and crew aboard the cruise ship Diamond Princess, homeless persons in Boston and Los Angeles, obstetric patients in New York City, and crew aboard the USS Theodore Roosevelt and Charles de Gaulle aircraft carrier, among several others. Approximately 40-45% of SARS-CoV-2 infections were asymptomatic. [45]
Utilizing a decision analytical model, Johansson et al from the US Centers for Disease Control and Prevention assessed transmission from presymptomatic, never symptomatic, and symptomatic individuals across various scenarios to determine the infectious period of transmitting SARS-CoV-2. Results from their base case determined 59% of all transmission came from asymptomatic transmission, comprising 35% from presymptomatic individuals and 24% from individuals who never develop symptoms. They estimate at least 50% of new SARSCoV-2 infections originated from exposure to individuals with infection, but without symptoms. [46]
Zou and colleagues followed viral expression through infection via nasal and throat swabs in a small cohort of patients. They found increases in viral loads at the time that the patients became symptomatic. One patient never developed symptoms but was shedding virus beginning at day 7 after presumed infection. [47]
Epidemiology
Coronavirus outbreak and pandemic
As of May 14, 2021, confirmed COVID-19 infections number over 160.8 million individuals worldwide and have resulted in over 3.3 million deaths. [48]
In the United States, over 32.6 million reported cases of COVID-19 have been confirmed as of May 14, 2021, resulting in over 581,000 deaths. [49] The pandemic caused approximately 375,000 deaths in the United States during 2020. The age-adjusted death rate increased by 15.9% in 2020, making it the third leading cause of death after heart disease and cancer. [50] Beginning in late March 2020, the United States had more confirmed infections than any other country in the world. [51] The United States also has the most confirmed deaths in the world, followed by Brazil, India, and Mexico. [48]
An interactive map of confirmed cases can be found here.
Health disparities
Communities of color have been disproportionally devastated by COVID-19 in the United States and in Europe. Data from New Orleans illustrated these disparities. African Americans represent 31% of the population but 76.9% of the hospitalizations and 70.8% of the deaths. [52]
The reasons are still being elucidated, but data suggest the cumulative effects of health disparities are the driving force. The prevalence of chronic (high- risk) medical conditions is higher and access to health care may be less available. Finally, socioeconomic status may decrease the ability to isolate and avoid infection. [53, 54]
Communities of color have been disproportionally devastated by COVID-19 in the United States and in Europe. Date from New Orleans illustrated these disparities. African Americans represent 31% of the population but 76.9% of the hospitalizations and 70.8% of the deaths.
The overall age-adjusted death rate increased by 15.9% in 2020. Death rates were highest among non-Hispanic Black persons and non-Hispanic American Indian or Alaska Native persons. [50]
CDC maintains a COVID-19 Data Tracker for near real time updates.
Young Adults
Outcomes from COVID-19 disease in young adults have been described by Cunningham and colleagues. Of 3200 adults aged 18-34 years hospitalized in the US with COVID-19, 21% were admitted to the ICU, 10% required mechanical ventilation, and 3% died. Comorbidities included obesity 33% (25% overall were morbidly obese), diabetes 18%, and hypertension 16%. Independent predictors of death or mechanical ventilation included hypertension, male gender, and morbid obesity. Young adults with multiple risk factors for poor outcomes from COVID-19 compared similarly to middle-aged adults without such risk factors. [55]
A study from South Korea found that older children and adolescents are more likely to transmit SARS CoV-19 to family members than are younger children. The researchers reported that the highest infection rate (18.6%) was in household contacts of patients with COVID-19 aged 10-19 years and the lowest rate (5.3%) was in household contacts of those aged 0-9 years. [56] Teenagers have been shown to be the source of clusters of cases illustrating the role of older children. [57]
COVID-19 in children
Data continue to emerge regarding the incidence and how children are affected by COVID-19, especially for severe disease. A severe multisystem inflammatory syndrome linked to COVID-19 infection has been described in children. [58, 59, 60, 61]
The American Academy of Pediatrics reports children represent 14% of all cases in the 49 states reporting by age, over 3.8 million children have tested positive in the United States since the onset of the pandemic as of May 6, 2021. This represents an overall rate of 5,122 cases per 100,000 children. During the 2-week period of April 22 to May 6, 2021 there was a 4% increase in cumulated number of children who tested positive, representing 143,716 new cases. In the week from 4/29/21-5/6/21, children represented 24% of the new weekly cases. Children were 1.2-3.1% of total reported hospitalizations, and between 0.1-1.9% of all child COVID-19 cases resulted in hospitalization. [62]
In September 2020, the CDC published the demographics of SARS-CoV-2-associated deaths among persons aged 21 years and younger. At the time of publication, approximately 6.5 million cases of SARS-CoV-2 infection and 190,000 associated deaths were reported in the United States. Persons younger than 21 years constitute 26% of the US population. Characteristics of the 121 COVID-related deaths among this population reported between February 12 to July 31, 2020 include [63] :
Male: 63%
Younger than 1 year: 10%
Aged 1-9 years: 20%
Aged 10-20 years: 70%
Hispanic: 45%
Black: 29%
Native American or Alaska persons: 4%
Underlying conditions: 75%
Died after hospital admission: 65%
Died at home or emergency department: 32%
Clinical characteristics and outcomes of hospitalized children and adolescents aged 1 month to 21 years with COVID-19 in the New York City area have been described. These observations alerted clinicians to rare, but severe illness in children. Of 67 children who tested positive for COVID-19, 21 (31.3%) were managed as outpatients. Among 46 hospitalized patients, 33 (72%) were admitted to the general pediatric medical unit and 13 (28%) to the pediatric intensive care unit (PICU). Obesity and asthma were highly prevalent, but not significantly associated with PICU admission (P = .99).
Admission to the pediatric intensive care unit (PICU) was significantly associated with higher C-reactive protein, procalcitonin, and pro-B type natriuretic peptide levels and platelet counts (P < .05 for all). Patients in the PICU were more likely to require high-flow nasal cannula (P = .0001) and were more likely to have received remdesivir through compassionate release (P < .05). Severe sepsis and septic shock syndromes were observed in 7 (53.8%) patients in the PICU. ARDS was observed in 10 (77%) PICU patients, 6 of whom (46.2%) required invasive mechanical ventilation for a median of 9 days. Of the 13 patients in the PICU, 8 (61.5%) were discharged home, and 4 (30.7%) patients remain hospitalized on ventilatory support at day 14. One patient died after withdrawal of life-sustaining therapy associated with metastatic cancer. [64]
A case series of 91 children who tested positive for COVID-19 in South Korea showed 22% were asymptomatic during the entire observation period. Among 71 symptomatic cases, 47 children (66%) had unrecognized symptoms before diagnosis, 18 (25%) developed symptoms after diagnosis, and 6 (9%) were diagnosed at the time of symptom onset. Twenty-two children (24%) had lower respiratory tract infections. The mean (SD) duration of the presence of SARS-CoV-2 RNA in upper respiratory samples was 17.6 (6.7) days. These results lend more data to unapparent infections in children that may be associated with silent COVID-19 community transmission. [65]
An Expert Consensus Statement has been published that discusses diagnosis, treatment, and prevention of COVID-19 in children.
Multisystem inflammatory syndrome in children
Media reports and a health alert from the New York State Department of Health drew initial attention to a newly recognized multisystem inflammatory syndrome in children (MIS-C) associated with COVID-19. Since then, MIS-C cases have been reported across the United States and Europe, and the American Academy of Pediatrics has published interim guidance.
Symptoms are reminiscent of Kawasaki disease, atypical Kawasaki disease, or toxic shock syndrome. All patients had persistent fevers, and more than half had rashes and abdominal complaints. Interestingly, respiratory symptoms were rarely described. Many patients did not have PCR results positive for COVID-19, but many had strong epidemiologic links with close contacts who tested positive. Furthermore, many had antibody tests positive for SARS-CoV-2. These findings suggest recent past infection, and this syndrome may be a postinfectious inflammatory syndrome. The CDC case definition requires:
An individual younger than 21 years presenting with fever ≥38.0°C for ≥24 hours, laboratory evidence of inflammation (including an elevated C-reactive protein [CRP], erythrocyte sedimentation rate [ESR], fibrinogen, procalcitonin, D-dimer, ferritin, lactic acid dehydrogenase [LDH], or interleukin 6 [IL-6], elevated neutrophils, reduced lymphocytes, and low albumin), and evidence of clinically severe illness requiring hospitalization, with multisystem (≥2) organ involvement (cardiac, renal, respiratory, hematologic, gastrointestinal, dermatologic, or neurological); AND
No alternative plausible diagnoses; AND
Positive for current or recent SARS-CoV-2 infection by RT-PCR, serology, or antigen test; or exposure to a suspected or confirmed COVID-19 case within the 4 weeks prior to the onset of symptoms.
Jiang and colleagues reviewed the literature on MIS-C noting the multiple organ system involvement. Unlike classic Kawasaki Disease, the children tended to be older and those of Asian ethnicity tended to be spared. [66]
A case series compared 539 patients who had MIS-C with 577 children and adolescents who had severe COVID-19. The patients with MIS-C were typically younger (predominantly aged 6-12 years) and more likely to be non-Hispanic Black. They were less likely to have an underlying chronic medical condition, such as obesity. Severe cardiovascular or mucocutaneous involvement was more common in those with MIS-C. Patients with MIS-C also had higher neutrophil to lymphocyte ratios, higher CRP levels, and lower platelet counts than those with severe COVID-19. [67]
COVID-19 in pregnant women and neonates
The US COVID-19 PRIORITY study (Pregnancy coRonavIrus Outcomes RegIsTrY) pregnancy registry is open; the study has a dashboard for real time data.
The CDC COVID-NET data published in September 2020 reported that among 598 hospitalized pregnant women with COVID-19, 55% were asymptomatic at admission. Severe illness occurred among symptomatic pregnant women, including intensive care unit admissions (16%), mechanical ventilation (8%), and death (1%). Pregnancy losses occurred for 2% of pregnancies completed during COVID-19-associated hospitalizations, and were experienced by both symptomatic and asymptomatic women. [68]
A multicenter study involving 16 Spanish hospitals reported outcomes of 242 pregnant women diagnosed with COVID-19 during the third trimester from March 13 to May 31, 2020. The women and their 248 newborns were monitored until the infant was 1 month old. Pregnant patients with COVID-19 who were hospitalized had a higher risk for cesarean birth (P = 0.027). Newborns whose mothers were hospitalized for COVID-19 infection had a higher risk for premature delivery (P = 0.006). No infants died and no vertical or horizontal transmission was detected. Exclusive breastfeeding was reported for 41.7% of infants at discharge and 40.4% at 1 month. [69]
A cohort study of pregnant women (n = 64) with severe or critical COVID-19 disease hospitalized at 12 US institutions between March 5, 2020, and April 20, 2020 rhas been published. At the time of the study, most women (81%) received hydroxychloroquine; 7% of women with severe disease and 65% with critical disease received remdesivir. All women with critical disease received either prophylactic or therapeutic anticoagulation. One case of maternal cardiac arrest occurred, but there were no cases of cardiomyopathy or maternal death. Half of the women (n = 32) delivered during their hospitalization (34% severe group; 85% critical group). Additionally, 88% with critical disease delivered preterm during their disease course, with 16 of 17 (94%) pregnant women giving birth through cesarean delivery. Overall, 15 of 20 (75%) women with critical disease delivered preterm. There were no stillbirths or neonatal deaths or cases of vertical transmission. [70]
Adhikari and colleagues published a cohort study evaluating 252 pregnant women with COVID-19 in Texas. Maternal illness at initial presentation was asymptomatic or mild in 95%of women, and 3% developed severe or critical illness. Compared with COVID negative pregnancies, there was no difference in the composite primary outcome of preterm birth, preeclampsia with severe features, or cesarean delivery for abnormal fetal heart rate. Early neonatal SARS-CoV-2 infection occurred in 6 of 188 tested infants,(3%) primarily born to asymptomatic or mildly symptomatic women. There were no placental pathologic differences by illness severity. [71]
Breastfeeding
A study by Chambers and colleagues found human milk is unlikely to transmit SARS-CoV-2 from infected mothers to infants. The study included 64 milk samples provided by 18 mothers infected with COVID-19. Samples were collected before and after COVID-19 diagnosis. No replication-competent virus was detectable in any of their milk samples compared with samples of human milk that were experimentally infected with SARS-CoV-2. [72]
Mothers who have been infected with SARS CoV-2 may have neutralizing antibodies expressed in breast milk. In an evaluation of 37 milk samples from 18 women, 76% contained SARS-CoV-2-specific IgA, and 80% had SARS-CoV-2-specific IgG. 62% of the milk samples were able to neutralize SARS-CoV-2 infectivity in vitro. These results support recommendations to continue breastfeeding with masking during mild-to-moderate maternal COVID-19 illness. [73]
COVID-19 in patients with HIV
Data for people with HIV and coronavirus are emerging. A multicenter registry has published outcomes for 286 patients with HIV who tested positive for COVID-19 between April 1 and July 1, 2020. Patient characteristics included mean age of 51.4 years, 25.9% were female, and 75.4% were African-American or Hispanic. Most patients (94.3%) were on antiretroviral therapy, 88.7% had HIV virologic suppression, and 80.8% had comorbidities. Within 30 days of positive SARS-CoV-2 testing, 164 (57.3%) patients were hospitalized, and 47 (16.5%) required ICU admission. Mortality rates were 9.4% (27/286) overall, 16.5% (27/164) among those hospitalized, and 51.5% (24/47) among those admitted to an ICU. [74]
Multiple case series have subsequently been published. Most suggest similar outcomes in patients living with HIV as the general patient population. [75, 76] Severe COVID-19 has been seen, however, suggesting that neither antiretroviral therapy of HIV infection are protective. [74, 77]
COVID-19 in clinicians
Among a sample of health care providers who routinely cared for COVID-19 patients in 13 US academic medical centers from February 1, 2020, 6% had evidence of previous SARS-CoV-2 infection, with considerable variation by location that generally correlated with community cumulative incidence. Among participants who had positive test results for SARS-CoV-2 antibodies, approximately one-third did not recall any symptoms consistent with an acute viral illness in the preceding months, nearly one half did not suspect that they previously had COVID-19, and approximately two-thirds did not have a previous positive test result demonstrating an acute SARS-CoV-2 infection. [78]
Prognosis
During January to December 2020, the estimated 2020 age-adjusted death rate increased for the first time since 2017, with an increase of 15.9% compared with 2019, from 715.2 to 828.7 deaths per 100,000 population. COVID-19 was the underlying or a contributing cause of 377,883 deaths (91.5 deaths per 100,000). COVID-19 death rates were highest among males, older adults, non-Hispanic American Indian or Alaska Native (AI/AN) persons, and Hispanic persons. Age-adjusted death rates was highest among Black (1,105.3) and AI/AN persons (1,024). [50]
COVID-19 deaths per 100,000 population in 2020 by age [50]
< 1 year: 1.1
1-4 years: 0.2
5-14 years: 0.2
15-24 years: 1.4
25-34 years: 5.5
35-44 years: 15.8
45-54 years: 44.2
55-64 years: 105.1
65-74 years: 249.2
75-84 years: 635.8
85 years or older: 1,797.8
Mortality and diabetes
Type 1 and type 2 diabetes are both independently associated with a significant increased odds of in-hospital death with COVID-19. A nationwide analysis in England of 61,414,470 individuals in the registry alive as of February 19, 2020, 0.4% had a recorded diagnosis of type 1 diabetes and 4.7% of type 2 diabetes. A total of 23,804 COVID-19 deaths in England were reported as of May 11, 2020, one-third were in people with diabetes, including 31.4% with type 2 diabetes and 1.5% with type 1 diabetes. Upon multivariate adjustment, the odds of in-hospital COVID-19 death were 3.5 for those with type 1 diabetes and 2.03 for those with type 2 diabetes, compared with deaths without known diabetes. Further adjustment for cardiovascular comorbidities found the odds ratios were still significantly elevated in both type 1 (2.86) and type 2 (1.81) diabetes. [79]
The CDC estimates diabetes is associated with a 20% increased odds of in-hospital mortality. [50]
Hospitalization and cardiometabolic conditions
O’Hearn et al estimate nearly 2 in 3 adults hospitalized for COVID-19 in the United States have associated cardiometabolic conditions including total obesity (BMI 30 kg/m2 or greater), diabetes mellitus, hypertension, and heart failure. [80]
Virology
The full genome of SARS-CoV-2 was first posted by Chinese health authorities soon after the initial detection, facilitating viral characterization and diagnosis. The CDC analyzed the genome from the first US patient who developed the infection on January 24, 2020, concluding that the sequence is nearly identical to the sequences reported by China. [1] SARS-CoV-2 is a group 2b beta-coronavirus that has at least 70% similarity in genetic sequence to SARS-CoV. [32] Like MERS-CoV and SARS-CoV, SARS-CoV-2 originated in bats. [1] A new virus variant emerges when the virus develops 1 or more mutations that differentiate it from the predominant virus variants circulating in a population. The CDC surveillance of SARS-CoV-2 variants includes US COVID-19 cases caused by variants. The site also includes which mutations are associated with particular variants. The CDC has launched a genomic surveillance dashboard and a website tracking US COVID-19 case trends caused by variants. Researchers are studying how variants may or may not alter the extent of protection by available vaccines. Mutations Viral mutations may naturally occur anywhere in the SARS-CoV-2 genome. Unlike the human DNA genome, which is slow to mutate, RNA viruses can readily, and quickly, mutate. A mutation may alter the viral function (eg, enhance receptor binding), or may have no discernable function. Mutations have been identified for the receptor-binding domain (RBD) on the spike protein of SARS-CoV-2. Several of these mutations display higher binding affinity to human ACE2, likely owing to enhanced structural stabilization of the RBD. Whether a mutation enhances viral transmission is a question to explore. Possible mechanisms of increased transmissibility include increased viral shedding, longer contagious interval, increased infectivity, or increased environmental stability. D614G mutation In early May 2020, a study by Korber and colleagues reported the emergence of a SARS-CoV-2 mutation (Spike D614G), one of several spike (S) mutations that have been discovered. [81] SARS-CoV-2 infections with this mutation have become the dominant viral lineage in North America, Europe, and Australia. The significance of the D614G mutation in terms of factors such as transmissibility, virulence, antigenicity, and potential treatment resistance is poorly understood. [82] Further research on the D614G spike protein mutation has now suggested a gain in fitness and transmission effectiveness. [83] An analysis of British data, the 614G mutation appeared to confer a selective advantage. There was no indication that patients infected with the Spike 614G variant have higher COVID-19 mortality or clinical severity, but 614G is associated with higher viral load and younger age of patients which could drive different transmission dynamics. [84] Mutations in the SARS CoV-2 spike protein RBD are being monitored as they have the potential to decrease the efficacy of neutralizing antibodies. Recent work has focused on the exposure to monoclonal antibody therapy driving selection of resistant mutants. [85] Viral variants that resist neutralization have been found circulating in the environment, [86] and have been selected by use of convalescent sera in a clinical setting. [87] Variants As mentioned, viruses such as SARS-CoV-2 change. Among the hundreds of variants detected in the first year of the pandemic, the ones that are most concerning are the so-called variants of concern (VOCs). Variants can affect efficacy of vaccines and antibody directed therapies. VOC – 202012/01 (SARS-CoV-2 lineage B.1.1.7) The CDC tracks variant proportions circulating in United States and estimates the B.1.1.7 variant (first detected in the United Kingdom) accounted for over 44% of cases from January 2 to March 27, 2021. On April 7, 2021, the CDC announced B.1.1.7 is the dominant strain circulating in the United States. A novel spike mutation with deletions of (delta)69/delta(70) has been shown to occur de novo on multiple occasions and be maintained through sustained transmission in association with other mutations. [88] This is the source of intense scrutiny in Europe, especially in the United Kingdom. A recent VOC – 202012/01 (201/501Y.V1) contains the deletion 69-70 as well as several other mutations including: N501Y, A570D, D614G, P681H, T716I, S982A, D1118H. The variant is being investigated as a cause of rapid increase in case numbers possibly due to increased viral loads and transmissibility. The N501Y mutation seems to increase viral loads 0.5 log. [89] Additionally, VOC-202012/01 has mutations that appear to account for its enhanced transmission. The N501Y replacement on the spike protein has been shown to increase ACE2 binding and cell infectivity in animal models. The deletion at positions 69 and 70 of the spike protein (delta69-70) has been associated with diagnostic test failure for the ThermoFisher TaqPath probe targeting the spike protein. Therefore, British labs are using this test failure to identify the variant. [90] Surveillance data from the UK national community testing (“Pillar 2”) showed a rapid increase in S-gene target failures (SGTF) in PCR testing for SARS-CoV-2 in November and December 2020. The R0 of this variant seems higher. At the same time that the transmission of the wild type virus was dropping, the variant increased, suggesting that the same recommendations (eg, masks, social distancing) may not be enough. The UK variant is also infecting more children (aged 19 years and younger) than the wild type indicating that it may be more transmissible in children. This has raised concerns because a relative sparing of children has been observed to date. This variant is hypothesized to have a stronger ACE binding than the original variant, which was felt to have trouble infecting younger individuals as they express ACE to a lesser degree. [90] Other VOCs Enhanced genomic surveillance in some countries have detected other VOCs including B.1.351 (501Y.V2) first detected in South Africa and the B.1.1.28 (renamed P.1) (501Y.V3) which was detected in 4 travelers from Brazil during routine screening at the Tokyo airport. [91] South African VOC The E484K mutation was found initially in the South Africa and Brazil variants in late 2020, and was observed in the UK variant in early February 2021. Position 484 and 501 mutations that are both present in the South African variant, and the combination is a concern that immune escape may occur. These mutations, among others, have combined to create the VOC B.1.351. The mutation at the 501 position changes the shape of the RBD by rotating it by 20 degrees to allow deeper binding. The mutation at the 484 position changes the RBD to a positive charge, and allows a higher affinity to the ACE2 receptor. [92] Brazilian VOCs Sabino et al describe resurgence of COVID-19 in Manaus, Brazil in January 2021, despite a high seroprevalence. A study of blood donors indicated that 76% of the population had been infected with SARS-CoV-2 by October 2020. Hospitalizations for COVID-19 in Manaus numbered 3,431 in January 1-19, 2021 compared with 552 for December 1-19, 2020. Hospitalizations had remained stable and low for 7 months prior to December. Several postulated variables regarding this resurgence include waning titers to the original viral lineage and the high prevalence of the P.1 variant, which was first discovered in Manaus. [93] In addition, researchers are monitoring emergence of a second variant in Brazil, P.2, identified in Rio de Janeiro. As of April 15, the CDC lists P.2 as a variant of interest. California VOCs VOCs B.1.427 and B.1.429 emerged in California. These variants accounted for 2.9% and 6.9% of variants circulating in the United States between January 2 to March 27, 2021. Researchers are studying how variants may or may not alter the extent of protection by available vaccines and antibody-directed therapies.
Clinical Presentation
History
Presentations of COVID-19 have ranged from asymptomatic/mild symptoms to severe illness and mortality. Common symptoms have included fever, cough, and shortness of breath. [13] Other symptoms, such as malaise and respiratory distress, have also been described. [32]
Symptoms may develop 2 days to 2 weeks after exposure to the virus. [13] A pooled analysis of 181 confirmed cases of COVID-19 outside Wuhan, China, found the mean incubation period to be 5.1 days and that 97.5% of individuals who developed symptoms did so within 11.5 days of infection. [14]
The following symptoms may indicate COVID-19 [16] :
Fever or chills
Cough
Shortness of breath or difficulty breathing
Fatigue
Muscle or body aches
Headache
New loss of taste or smell
Sore throat
Congestion or runny nose
Nausea or vomiting
Diarrhea
Other reported symptoms have included the following:
Sputum production
Malaise
Respiratory distress
Neurologic (eg, headache, altered mentality)
Wu and McGoogan reported that, among 72,314 COVID-19 cases reported to the Chinese Center for disease Control and Prevention (CCDC), 81% were mild (absent or mild pneumonia), 14% were severe (hypoxia, dyspnea, >50% lung involvement within 24-48 hours), 5% were critical (shock, respiratory failure, multiorgan dysfunction), and 2.3% were fatal. [15] These general symptom distribution have been reconfirmed across multiple observations. [94, 95]
Clinicians evaluating patients with fever and acute respiratory illness should obtain information regarding travel history or exposure to an individual who recently returned from a country or US state experiencing active local transmission. [96]
Williamson and colleagues, in an analysis of 17 million patients, reaffirmed that severe COVID-19 and mortality was more common in males, older individuals, individuals in poverty, Black persons, and patients with medical conditions such as diabetes and severe asthma, among others. [97]
A multicenter observational cohort study conducted in Europe found frailty to be a greater predictor of mortality than age or comorbidities. [98]
Type A blood has been suggested as a potential factor that predisposes to severe COVID-19, specifically in terms of increasing the risk of respiratory failure. Blood type O appears to confer a protective effect. [99, 100]
Patients with suspected COVID-19 should be reported immediately to infection-control personnel at their healthcare facility and the local or state health department. Current CDC guidance calls for the patient to be cared for with airborne and contact precautions (including eye shield) in place. [19] Patient candidates for such reporting include those with fever and symptoms of lower respiratory illness who have travelled from Wuhan City, China, within the preceding 14 days or who have been in contact with an individual under investigation for COVID-19 or a patient with laboratory-confirmed COVID-19 in the preceding 14 days. [96]
A complete or partial loss of the sense of smell (anosmia) has been reported as a potential history finding in patients eventually diagnosed with COVID-19. [17] A phone survey of outpatients with mildly symptomatic COVID-19 found that 64.4% (130 of 202) reported any altered sense of smell or taste. [18] In a European study of 72 patients with PCR results positive for COVID-19, 53 patients (74%) reported reduced olfaction, while 50 patients (69%) reported a reduced sense of taste. Forty-nine patients (68%) reported both symptoms. [101]
Physical Examination
Patients who are under investigation for COVID-19 should be evaluated in a private room with the door closed (an airborne infection isolation room is ideal) and asked to wear a surgical mask. All other standard contact and airborne precautions should be observed, and treating healthcare personnel should wear eye protection. [19]
The most common serious manifestation of COVID-19 upon initial presentation is pneumonia. Fever, cough, dyspnea, and abnormalities on chest imaging are common in these cases. [102, 103, 104, 105]
Huang and colleagues found that, among patients with pneumonia, 99% had fever, 70% reported fatigue, 59% had dry cough, 40% had anorexia, 35% experienced myalgias, 31% had dyspnea, and 27% had sputum production. [102]
Complications
Complications of COVID-19 have included pneumonia, acute respiratory distress syndrome, cardiac injury, arrhythmia, septic shock, liver dysfunction, acute kidney injury, and multi-organ failure, among others. Approximately 5% of patients with COVID-19, and 20% of those hospitalized, experience severe symptoms necessitating intensive care. The common complications among hospitalized patients include pneumonia (75%), ARDS (15%), AKI (9%), and acute liver injury (19%). Cardiac injury has been increasingly noted including troponin elevation, acute heart failure, dysrhythmias, and myocarditis. 10-25% of hospitalized COVID-19 patients experience prothrombotic coagulopathy resulting in venous and arterial thromboembolic events. Neurologic manifestations include impaired consciousness and stroke. ICU case fatality is reported up to 40%. [94] Post-acute Sequelae of SARS-CoV-2 Infection As the COVID-19 pandemic has matured, more patients have reported long-term, post infection sequelae. The majority of patients recover fully but those that do not have reported adverse symptoms such as fatigue, dyspnea, cough, anxiety, depression, inability to focus (ie, “brain fog”), gastrointestinal problems, sleep difficulties, joint pain, and chest pain lasting weeks to months after the acute illness. Long-term studies are underway to understand the nature of these complaints. [106] Post-acute sequelae of SARS-CoV-2 (PASC) infection is the medical term for what is commonly called ‘long COVID’ (or Long Haulers). The US National Institutes of Health includes discussion of persistent symptoms or organ dysfunction after acute COVID-19 within guidelines that discuss the clinical spectrum of the disease. [107] The UK National Institute for Health and Care Excellence (NICE) issued guidelines on care of long-COVID that define the syndrome as: signs and symptoms that develop during or after an infection consistent with COVID-19, continue for more than 12 weeks, and are not explained by an alternative diagnosis. [108] An international web-based survey of respondents (n = 3,762) with suspected and confirmed COVID-19 from 56 countries tallied prevalence of 205 symptoms in 10 organ systems, with 66 symptoms traced over 7 months. The most frequent symptoms reported after 6 months were fatigue (77.7%), postexertional malaise (72.2%), and cognitive dysfunction (55.4%). Nearly 50% were unable to return to work 6 months after infection. [109] A long-term follow-up study of adults with non-critical COVID-19 at 30 and 60 days post infection revealed ongoing symptoms in two-thirds of patients. The most common symptoms included anosmia/ageusia in 28% (40/150) at day 30 and 23% (29/130) at day 60; dyspnea in 36.7% (55/150) patients at day 30 and 30% (39/130) at day 60; and fatigue/weakness in 49.3% (74/150) at day 30 and 40% (52/130) at day 60. Persistent symptoms at day 60 were significantly associated with age 40 to 60 years old, hospital admission, and abnormal auscultation at symptom onset. [110] A follow-up study of COVID-19 consequences in 1,733 patients discharged from the hospital in Wuhan, China after 6 months reported fatigue or muscle weakness (63%), sleep difficulties (26%), and anxiety or depression (23%) were the most common symptoms. Lung function, as measured by CT showing interstitial change and 6-minute walking distance, was less than the lower limit of normal for 22-56% across different severity scales. [111] A study of 55 patients from China looked at long-term pulmonary follow-up 3 months after discharge from a symptomatic COVID-19 illness. Patients’ mean age was 47 years, 42% were female, and 85% had moderate disease. Only 9 patients (16.4%) had underlying comorbidities including hypertension, diabetes mellitus, and cardiovascular diseases, but none had preexisting pulmonary disease. None of the patients required mechanical ventilation. At 3 months, 71% still had abnormal chest CT scans, most commonly showing interstitial thickening. Spirometry was also checked in all patients. Lung function abnormalities were detected in 25.5%. Anomalies were noted in total lung capacity of 4 patients (7.3%), FEV1 of 6 patients (11%), FVC of 6 patients (11%), DLCO of 9 patients (16%), and small airway function in 7 patients (12%) despite most patients having no respiratory complaints. [112] These data are consistent with the findings of a study of 124 patients recovered from COVID-19 after 6 weeks in the Netherlands. The mean age was 59±14 years and 60% were male; 27 with mild, 51 with moderate, 26 with severe, and 20 with critical disease. Nearly all patients (99%) had improved imaging, but residual parenchymal abnormalities remained in 91% and correlated with reduced lung diffusion capacity in 42%. Twenty-two percent had low exercise capacity, 19% low fat-free mass index, and problems in mental and/or cognitive function were found in 36% of the patients. [113] The long-term effects of COVID-19 have also been observed after mild infection treated in the outpatient setting. In a longitudinal cohort study at the University of Washington, 177 participants completed a survey a median of 169 days after their COVID-19 diagnosis. Almost 85% were never admitted for treatment. One third reported persistent symptoms, and a similar number reported worsened quality of life. The most common symptom was fatigue. [114] Future public health implications Public health implications for PASC need to be examined, as reviewed by Datta, et al. As with other infections (eg, Lyme disease, syphilis, Ebola), late inflammatory and virologic sequelae may emerge. Accumulation of evidence beyond the acute infection and postacute hyperinflammatory illness is important to evaluate to gain a better understanding of the full spectrum of the disease. [115] Thrombotic manifestations of severe COVID-19 are caused by the ability of SARS-CoV-2 to invade endothelial cells via angiotensin-converting enzyme-2 (ACE-2), which is expressed on the surface of endothelial cells. Subsequent endothelial inflammation, complement activation, thrombin generation, platelet, and leukocyte recruitment, and the initiation of innate and adaptive immune responses culminate in immunothrombosis, and can ultimately cause microthrombotic complications (eg, DVT, PE, stroke). [116] Kotecha et al describe patterns of myocardial injury in hospitalized patients with severe COVID-19 who had elevated troponin levels. During convalescence, myocarditis-like injury was observed, with limited extent and minimal functional consequence. However, in a proportion of patients, there was evidence of possible ongoing localized inflammation. Roughly 25% of patients had ischemic heart disease, of which two-thirds had no previous history. [117] Reinfection Clinicians, infectious disease specialists, and public health experts are examining the potential for patient reinfection with the SARS CoV-2 virus. [118] Cases of reinfection with SARS CoV-2 have emerged worldwide. [119] Several cases have shown differing viral genomes tested in the patient, which suggests reinfection rather than prolonged viral shedding. A case report showed a 42-year-old male who was infected with SARS CoV-2 on March 21, 2020 following a workplace exposure. The patient had resolution of symptoms after 10 days with continued good health for 51 days. On May 24, 2020, the patient presented with symptoms suggestive of COVID-19 following a new household exposure. Upon testing via SARS-CoV-2 RT-PCR, the patient had confirmed positive COVID-19 with several potential genetic variations that differed from the SARS-CoV-2 strain sequenced from the patient in March. [120] In another case, a 33-year-old male in Hong Kong had contracted COVID-19 in March 2020, which was confirmed via saliva SARS-CoV-2 RT-PCR. The patient had resolution of symptoms along with two negative SARS-CoV-2 RT-PCR results by April 14, 2020. The patient experienced a second episode of COVID-19 in August 2020 following a trip to Spain. Although asymptomatic, the patient was tested upon returning to Hong Kong and tested positive via SARS-CoV-2 RT-PCR. Genomic sequencing was performed on both RT-PCR specimens collected in March and August. The genomic analysis showed the two strains of SARS-CoV-2 (from March and August) belonged to different viral lineages, which suggests that the strain from the first episode differed from the strain in the second episode. [121] The Collaborative Study COVID Recurrences (COCOREC) group in France reported 11 virologically-confirmed cases of patients with a second clinically- and virologically confirmed acute COVID-19 episodes between April 6, 2020 and May 14, 2020. Although, the letter does not describe confirmation with viral genomic sequencing to understand if the cases were a relapse of the initial infection or a new infection. [122] Two cases of reinfection have emerged in the United States, a 25-year-old man from Nevada and a 42-year-old man in Virginia. These cases were confirmed by gene testing that showed different strains of the SARS-CoV-2 virus during the 2 infection episodes in each patient. In these cases, the patients experienced more severe symptoms during their second infections. It is unclear if the symptom severity experienced the second time were related to the virus or the how the patients’ immune systems reacted. Vaccine development may need to take into account circulating viral strains. [119, 123] These case reports give insight to the possibility of reinfection. Further research to determine the prevalence of COVID-19 reinfections is needed, including the frequency at which they occur and longevity of COVID-19 immunity.
Workup
Approach Considerations
Diagnostic testing for SARS-CoV-2 infection can be conducted by the CDC, state public health laboratories, hospitals using their own developed and validated tests, and some commercial reference laboratories. [124]
State health departments with a patient under investigation (PUI) should contact CDC’s Emergency Operations Center (EOC) at 770-488-7100 for assistance with collection, storage, and shipment of clinical specimens for diagnostic testing. Specimens from the upper respiratory tract, lower respiratory tract, and serum should be collected to optimize the likelihood of detection. [96]
The FDA now recommends that nasal swabs that access just the front of the nose be used in symptomatic patients, allowing for (1) a more comfortable and simplified collection method and (2) self-collection at collection sites. [125]
Various organizations, including the CDC, have published guidelines on COVID-19.
Laboratory Studies
Signs and symptoms of coronavirus disease 2019 (COVID-19) may overlap with those of other respiratory infections; therefore, it is important to perform laboratory testing to specifically identify symptomatic individuals infected with severe acute respiratory syndrome coronavirus 2 (SARS-CoV-2).
There are 3 types of tests that may be utilized to determine if an individual has been infected with SARS-CoV-2:
Viral nucleic acid (RNA) detection
Viral antigen detection
Detection of antibodies to the virus
Viral tests (nucleic acid or antigen detection tests) are used to assess acute infection, whereas antibody tests provide evidence of prior infection with SARS-CoV-2. Home sample collection kits for COVID-19 testing have been available by prescription, in December 2020, the LabCorp Pixel COVID-19 Test Home Collection Kit became the first to receive an FDA EUA for nonprescription use.
The FDA has advised against the use of antibody tests to ascertain immunity or protection from COVID-19, particularly in patients who have been vaccinated against the disease. According to the agency, differences between antibodies that arise from prior SARS-CoV-2 infection and those induced by vaccination leave the tests unable to determine whether an individual has achieved protection through a vaccine.
Laboratory findings in patients with COVID-19
Leukopenia, leukocytosis, and lymphopenia were common among early cases. [32, 102]
Lactate dehydrogenase and ferritin levels are commonly elevated. [102]
Wu and colleagues [126] reported that, among 200 patients with COVID-19 who were hospitalized, older age, neutrophilia, and elevated lactate dehydrogenase and D-dimer levels increased the risks of ARDS and death.
CT Scanning
Chest computed tomography scanning in patients with COVID-19–associated pneumonia usually shows ground-glass opacification, possibly with consolidation. Some studies have reported that abnormalities on chest CT scans are usually bilateral, involve the lower lobes, and have a peripheral distribution. Pleural effusion, pleural thickening, and lymphadenopathy have also been reported, although with less frequency. [102, 127, 128]
Bai and colleagues reported the following common chest CT scanning features among 201 patients with CT abnormalities and positive RT-PCR results for COVID-19 [129] :
Peripheral distribution (80%)
Ground-glass opacity (91%)
Fine reticular opacity (56%)
Vascular thickening (59%)
Less-common features on chest CT scanning included the following [129] :
Central and peripheral distribution (14%)
Pleural effusion (4.1%)
Lymphadenopathy (2.7%)
The American College of Radiology (ACR) recommends against using CT scanning for screening or diagnosis but instead reserving it for management in hospitalized patients. [130]
At least two studies have reported on manifestations of infection in apparently asymptomatic individuals. Hu and colleagues reported on 24 asymptomatic infected persons in whom chest CT scanning revealed ground-glass opacities/patchy shadowing in 50% of cases. [131] Wang and colleagues reported on 55 patients with asymptomatic infection, two-thirds of whom had evidence of pneumonia as revealed by CT scanning. [132]
Progression of CT abnormalities
Li and colleagues recommend high-resolution CT scanning and reported the following CT changes over time in patients with COVID-19 among 3 Chinese hospitals:
Early phase: Multiple small patchy shadows and interstitial changes begin to emerge in a distribution beginning near the pleura or bronchi rather than the pulmonary parenchyma.
Progressive phase: The lesions enlarge and increase, evolving to multiple ground-glass opacities and infiltrating consolidation in both lungs.
Severe phase: Massive pulmonary consolidations occur, while pleural effusion is rare.
Dissipative phase: Ground-glass opacities and pulmonary consolidations are absorbed completely. The lesions begin evolving into fibrosis. [133]
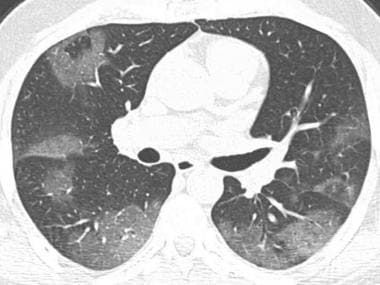
Axial chest CT demonstrates patchy ground-glass opacities with peripheral distribution.
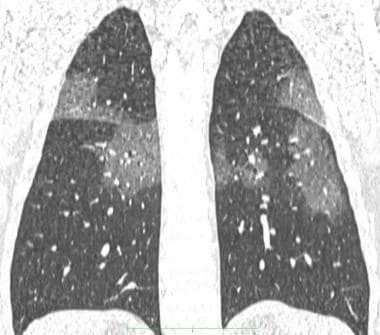
Coronal reconstruction chest CT of the same patient above, showing patchy ground-glass opacities.
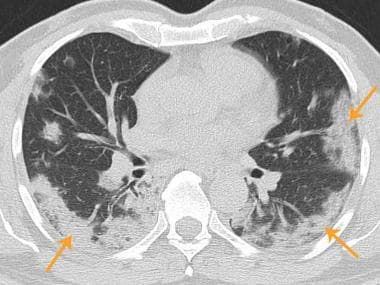
Axial chest CT shows bilateral patchy consolidations (arrows), some with peripheral ground-glass opacity. Findings are in peripheral and subpleural distribution.
Chest Radiography
In a retrospective study of patients in Hong Kong with COVID-19, common abnormalities on chest radiography, when present, included consolidation (30 of 64 patients; 47%) and ground-glass opacities (33%). Consolidation was commonly bilateral and of lower zone distribution. Pleural effusion was an uncommon finding. Severity on chest radiography peaked 10-12 days after system onset. [134]
Chest radiography may reveal pulmonary infiltrates. [135]

The heart is normal in size. There are diffuse, patchy opacities throughout both lungs, which may represent multifocal viral/bacterial pneumonia versus pulmonary edema. These opacities are particularly confluent along the periphery of the right lung. There is left midlung platelike atelectasis. Obscuration of the left costophrenic angle may represent consolidation versus a pleural effusion with atelectasis. There is no pneumothorax.
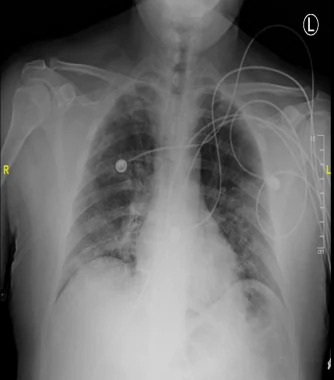
The heart is normal in size. There are bilateral hazy opacities, with lower lobe predominance. These findings are consistent with multifocal/viral pneumonia. No pleural effusion or pneumothorax are seen.
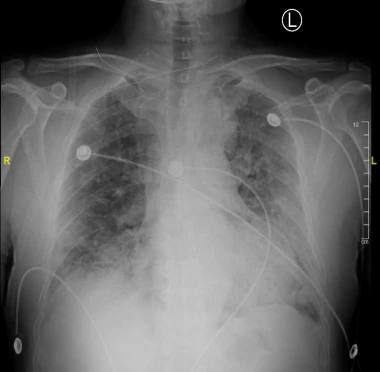
The heart is normal in size. Patchy opacities are seen throughout the lung fields. Patchy areas of consolidation at the right lung base partially silhouettes the right diaphragm. There is no effusion or pneumothorax. Degenerative changes of the thoracic spine are noted

The same patient as above 10 days later.

The trachea is in midline. The cardiomediastinal silhouette is normal in size. There are diffuse hazy reticulonodular opacities in both lungs. Differential diagnoses include viral pneumonia, multifocal bacterial pneumonia or ARDS. There is no pleural effusion or pneumothorax.
Comments